Launch the Next Nuclear Corps for a More Flexible Nuclear Regulatory Commission
The Nuclear Regulatory Commission (NRC), the Nation’s regulator of civilian nuclear technologies, should shift agency staff, resources, and operations more flexibly based on emergent regulatory demands. The nuclear power industry is demonstrating commercialization progress on new reactor concepts that will challenge the NRC’s licensing and oversight functions. Rulemaking on new licensing frameworks is in progress, but such regulation will fall short without changes to the NRC’s staffing. Since the NRC is exempt from civil service laws under the Atomic Energy Act (AEA) of 1954, the agency should use AEA flexible hiring authorities to launch the Next Nuclear Corps, a staffing program to shift capacity based on emergent, short-term workforce needs. The NRC should also better enable hiring managers to meet medium-term workforce needs by clarifying guidance on NRC’s direct hire authority.
Challenge and Opportunity
Policymakers, investors, and major energy users, such as data centers and industrial plants, are interested in new nuclear power because it promises unique value. New nuclear power technologies could add either additional base load or variable power to electrical systems. Small modular or micro reactors could provide independent power to military bases, many of which are connected to power grids and vulnerable to disruption. Local governments can stimulate economies with high-paying and safe jobs at nuclear plants. The average nuclear power plant also has the lowest lifecycle greenhouse gas emissions compared to other available electricity-generating technologies, including wind, solar, and hydropower. Current efforts to expand nuclear power are different from those of the 1970s and 1980s, the most recent decades of significant building. Proposals today include building plants designed similarly to plants of those decades or even restarting power operations at up to three closed plants; but more activity is focused on commercializing advanced and small modular reactors, diverse concepts incorporating innovations in reactor design, fuel types, and safety systems. The government has partnered with private companies to develop and demonstrate advanced reactors since the inception of nuclear technology in the 1950s, but today several companies demonstrate advanced technical and business progress toward commercialization.
Innovation in nuclear power challenges the NRC’s status-quo approaches to licensing and oversight. Rulemaking on new regulatory frameworks is necessary and in progress, but changes to the agency’s staffing and operations are also needed. Over time, Congress, the President, and the Commission itself have adjusted the agency’s operations in response to shifts in international postures, comprehensive national energy plans, and accidents or emerging threats at nuclear plants, but the NRC’s ability to respond to sudden changes in the nuclear industry is a long-standing challenge. To become more flexible, NRC initiated Project Aim in 2014 after expectations of significant industry growth, spurred in part by tax incentives in the Energy Policy Act of 2005, were not realized due to record-low natural gas prices. More recent assessments from the Government Accountability Office (GAO) and NRC Office of Inspector General (OIG) acknowledge the challenge of workload forecasting in an unpredictable nuclear industry, but counterintuitively, some recommendations focus on improving the ability to workforce plan two years or more in advance. Renewed expectations of growth, spurred by interest from policymakers and energy customers, reinforces a point from the 2015 Project Aim final report that, “…effectiveness, efficiency, agility, flexibility, and performance must improve for the agency to continue to succeed in the future.”
Congress also called on the NRC to become more responsive to current developments as expressed in legislation enacted with bipartisan support. Across the Fiscal Responsibility Act of 2023 and the ADVANCE Act of 2024, Congress requires the NRC to update its mission statement to better reflect the benefits of civilian nuclear technology, establish regulatory frameworks for new technology, streamline environmental review, incentivize licensing of advanced nuclear technologies, and position itself and the United States as a leader in civilian nuclear power. Meeting expectations requires significant operational and workforce changes. Since NRC is exempt from civil service laws and operates an independent competitive merit system, widespread changes to the agency’s hiring practices will be determined by future Commissioners, including the President’s selection of Chair (and by extension, the Chair’s selection of the Executive Director for Operations (EDO)), and modifications to agreements between the NRC and the Office of Personnel Management (OPM). In the meantime, NRC is well equipped to increase hiring flexibility using authorities from existing law and regulations.
Plan of Action
Recommendation 1. The NRC EDO should launch the Next Nuclear Corps, a staffing program dedicated to shifting agency capacity based on short-term workforce needs.
The EDO should hire a new director to lead the Corps. The Corps director should report to the EDO and consult with the Office of the Chief Human Capital Officer (OCHCO) and division heads to develop Corps positions to address near-term priorities in competency areas that do not require in-depth training. Near-term priorities should be informed by the NRC’s existing yearly capacity assessments, but the Corps director should also rely on direct expertise and insights from branch chiefs who have a real-time understanding of industry activity and staffing challenges.
Recommendation 2. Hiring for the Corps should be executed under the special authority to appoint directly to the excepted service under 161B(a) of the Atomic Energy Act (AEA).
The ADVANCE Act of 2024 created new categories of hires to fill critical needs related to licensing, oversight, and matters related to NRC efficiency. The EDO should execute the Corps under the new authorities in section 161B(a) of the AEA as it provides clear direction and structure for the EDO to make personnel appointments outside of the NRC’s independent competitive merit system described in Management Directive 10.1. 161B(a)(A) provides up to 210 hires at any time and 161B(a)(B) provides up to 20 additional hires each fiscal year which are limited to a term of four years. The standard service term should be one year as near-term workforce needs may be temporary because of the nature of the position or uncertainty in future demand.
The EDO should adopt the following practices to allow renewals of some positions from the prior year without reaching the limits described in the AEA:
- 161B(a)(A): Appoint up to 140 new staff each fiscal year and consider staggering appointments to address capacity needs that arise later in the year. After the initial one-year term, up to half of the positions should be eligible for a one-year renewal if the need continues. After the initial cohort off-boards, an additional 140 new staff should be appointed alongside up to 70 renewed staff from the prior cohort without exceeding the maximum of 210 appointments at any time.
- 161B(a)(B): Appoint up to 20 new staff each fiscal year and consider staggering appointments to address capacity needs that arise later in the year. All positions should be eligible for a one-year renewal for up to three additional years if the need continues.
Recommendation 3. The EDO should update Management Directives 10.13 and 10.1 to contain or reference the standard operating procedure for NRC’s mirrored version of OPM’s Direct Hire Authority.
The proposed Corps addresses emergent, short-term capacity needs, but internal policy clarity is needed to solve medium-term hiring challenges for hard-to-recruit positions. As far back as 2007, NRC hiring managers and human resources reported that DHA was highly desired for hiring flexibility. The NRC OIG closed Recommendation 2.1 from Audit of the U.S. Nuclear Regulatory Commission’s Vacancy Announcement Process in June 2024 because NRC updated Standard Operating Procedure for Direct Hire Authority with more details. However, management directives are the primary policy and procedure documents that govern the NRC’s internal functions. The EDO should update management directives to formally capture or reference this procedure so that NRC staff are better equipped to use DHA. Specifically, the EDO should:
- amend Management Directive 10.13 Special Employment Programs to add Section IX. Direct Hire Authority, that formalizes the procedure in the Standard Operating Procedure for Direct Hire Authority
- update Management Directive 10.1, Section I.A. to reference the amended Management Directive 10.13 as the general policy for non-competitive hiring
Conclusion
The potential of new nuclear power plants to meet energy demand, increase energy security, and revitalize local economies depends on new regulatory and operational approaches at the NRC. Rulemaking on new licensing frameworks is in progress, but the NRC should also use AEA flexible hiring authorities to address emergent, short-term workforce needs that may be temporary based on shifting industry developments. The proposed Corps structure allows the EDO to quickly hire new staff outside of the agency’s competitive merit system for short-term needs while preserving flexibility to renew appointments if the capacity needs continue. For permanent hard-to-recruit positions, the EDO should clarify guidance for hiring managers on direct hire authority. The NRC is well equipped with existing authorities to meet emergent regulatory demand and renewed expectations of nuclear power growth.
This action-ready policy memo is part of Day One 2025 — our effort to bring forward bold policy ideas, grounded in science and evidence, that can tackle the country’s biggest challenges and bring us closer to the prosperous, equitable and safe future that we all hope for whoever takes office in 2025 and beyond.
PLEASE NOTE (February 2025): Since publication several government websites have been taken offline. We apologize for any broken links to once accessible public data.
The Corps director should create positions informed by the expertise and insights from agency leaders who have a real-time understanding of industry activity and present staffing challenges. Positions should cover all career levels and cover competency areas that do not require in-depth internal training or security clearances. The Corps should fill new positions created for special roles in support of other staff or teams, such as special coordinators, specialists, and consultants.
The Corps is not a graduate-level fellowship or leadership development program. The Corps is specifically for short-term, rapid hiring based on emergent capacity needs that may be temporary based on the nature of the need or uncertainty in future demand.
The Corps structure includes flexibility for a limited number of renewals, but it is not intended to recruit for permanent positions. Supervisors and hiring managers could choose to coordinate with the OCHCO to recruit off-boarding Corps members to other employment opportunities.
The Corps director can identify talent through existing NRC recruiting channels, such as job fairs, universities, and professional associations, however, the Corps director should also establish new recruiting efforts through more competitive channels. Because the positions are temporary, the Corps can recruit from more competitive talent pools, such as talent seeking long term careers in private industry. Job seekers with long-term ambitions in the private nuclear sector and the NRC could both benefit from a one- or two-year period of service focused on a specific project.
Promoting Fusion Energy Leadership with U.S. Tritium Production Capacity
As a fusion energy future becomes increasingly tangible, the United States should proactively prepare for it if/when it arrives. A single, commercial-scale fusion reactor will require more tritium fuel than is currently available from global civilian-use inventories. For fusion to be viable, greater-than-replacement tritium breeding technologies will be essential. Before the cycle of net tritium gain can begin, however, the world needs sufficient tritium to complete R&D and successfully commission first-of-a-kind (FOAK) fusion reactors. The United States has the only proven and scalable tritium production supply chain, but it is largely reserved for nuclear weapons. Excess tritium production capacity should be leveraged to ensure the success of and U.S. leadership in fusion energy.
The Trump administration should reinforce U.S. investments and leadership in commercial fusion with game changing innovation in the provision of tritium fuel. The Congressional Fusion Energy Caucus has growing support in the House with 92 members and an emerging Senate counterpart chaired by Sen. Martin Heinrich. Energy security and independence are important areas of bipartisan cooperation, but strong leadership from the White House will be needed to set a bold, America-first agenda.
Challenge and Opportunity
Fusion energy R&D currently relies on limited reserves of tritium from non-scalable production streams. These reserves reduce by ~5% each year due to radioactive decay, which makes stockpiling difficult. One recent estimate suggests that global stocks of civilian-use tritium are just 25–30kg, while commissioning and startup of a single commercial fusion reactor may require up to 10kg. The largest source of civilian-use tritium is Canada, which produces ~2kg/yr as a byproduct of heavy water reactor operation, but most of that material is intended to fuel the International Thermonuclear Experimental Reactor (ITER) in the next decade. This tritium production is directly coupled to the power generation rate of its fleet of Canadian Deuterium Uranium (CANDU) reactors; therefore, the only way to increase the tritium production rate is to build more CANDU power reactors.
The National Nuclear Security Administration (NNSA) (an Office of the U.S. Department of Energy (DOE)) – in cooperation with the Tennessee Valley Authority (TVA) – will produce up-to ~4kg of tritium over the next fuel cycles (i.e., ~18-month cycles offset by 6 months) for the two Watts Bar nuclear (WBN) reactors. This would exceed the current, combined 2.8kg production goal, which could be further outstripped if the reactors were operated at their maximum licensed limit, producing ~4.7kg of tritium. All this tritium is designated for military use. However, the NNSA and DOE could leverage production capacities in excess of defense requirements to promote the deployment of FOAK reactors and support U.S. leadership in fusion energy. The DOE could build off the success of its current Milestone-Based Fusion Program by integrating the option for additional tritium availability to meet the commissioning demands of pilot and commercial fusion reactors.
This program could be called “Gigatons-to-Gigawatts” (GtG), a name inspired by one of the most successful fissile material reduction programs in history Megatons-to-Megawatts. The increased scale signifies much higher energy densities contained in tritium vs. the uranium commonly used to fuel fission reactors. Fusion and fission reactor technologies also have very different nonproliferation implications. U.S. national security and nonproliferation goals would be furthered by a systematic transition from fission to fusion energy. Lowering reliance on dual-use nuclear fuel cycle technologies such as centrifuges for uranium enrichment would lower overall proliferation risks. Just as it did by promoting an open fuel cycle, the United States could leverage its technological leadership to promote the adoption of a more proliferation-resistant fusion infrastructure.
However, it is important to note another key difference with Megatons-to-Megawatts: because GtG leverages near-term tritium production capacities in concert with reserves rather than repurposing stockpiled weapons-useable material for civilian use such a program could affect the U.S. nuclear deterrent posture as well. The National Nuclear Security Administration (NNSA) Strategic Integrated Roadmap highlights the goal to “Demonstrate enhanced tritium production capability” for 2025 which is coded as “Nuclear Deterrent.” The anticipated excess production quantities noted above would correspond with this goal. Starting from this demonstrated capability, a GtG program would extend this production capacity into a longer-term effort directed toward a fusion energy future. Furthermore, in support of the long-term goal of nuclear disarmament, GtG would also provide a ready-made framework for repurposing valuable tritium from decommissioned warheads.
One way the United States demonstrates the credibility of its nuclear deterrent is through the Stockpile Stewardship and Management Plan (SSMP). Allies and adversaries alike must believe that the United States has sufficient tritium capability to replenish this critical and slowly decaying resource. An enhanced tritium production capability also has a supporting role to play in reassuring U.S. policymakers that key material design requirements are being sustainably met and that future nuclear weapon tests will be unnecessary. Even though GtG would be programmatically dedicated to the peaceful use of tritium, the technological mechanisms used to reach this goal would nonetheless be compatible with and/or even complementary to the existing nuclear defense posture.
Key facts highlighted in the 2024 Fusion Industry Association (FIA) global reports include: (i) tritium remains the key fuel source for most fusion technologies being developed; (ii) tritium self-sufficiency was seen as one of the major near-term challenges and by a slim margin the major challenge after 2030; and (iii) supply chain partners noted tritium was one of the top 3 constraints to scalability. The easiest reaction to achieve is deuterium–tritium (D–T) fusion. Other more technologically challenging approaches to fusion energy rely on different reactions such as deuterium–deuterium (D–D) and deuterium–Helium-3 (D–He-3) fusion. The Earth has a functionally limitless supply of deuterium; however, even though He-3 is radioactively stable, it slowly leaks from the atmosphere into space. Until humanity can mine the vast quantities of He-3 on the moon, one of the only terrestrial sources of this material is from the tritium decay process. A GtG program would directly support an increase in tritium supply and indirectly support long-term He-3 reserves since it can be stockpiled. Even if fusion with He-3 proves viable, it will be necessary to produce the tritium first.
Once commercial fusion reactors begin operation, breeding tritium to replace burned fuel is a major concern because there is no alternative supply sufficient to replace shortfalls from even modest inefficiency. Operating a 1 GW fusion reactor for a year may require more than 55kg of tritium. Tritium self-sufficiency is nonnegotiable for a functional fusion industry. If technological development falters as companies strive toward a sustainable tritium breeding cycle, they may find themselves in the awkward position of needing tritium more than additional funding.
Of the countries leading the way in private fusion ventures and public investment, the only not closely allied with the U.S. is China, which is also the country most capable of leveraging military tritium production for fusion R&D. In stark contrast with the United States, there is no public information on Chinese tritium production capacities or how much they currently possess. Since China is rapidly expanding their nuclear weapon stockpile, their material margins for repurposing tritium for peaceful-use material will be constrained. If a U.S. investment of tritium into fusion R&D accelerates the growth of domestic companies, then China may be forced to choose between advancing their nuclear weapons agenda and competing with the West for a fusion energy breakthrough.
The United States already has a significant lead in technological capabilities for future generations of fusion energy based on Inertial Confinement Fusion (ICF). The National Ignition Facility (NIF) at Lawrence Livermore National Labs (LLNL) first demonstrated fusion ignition from ICF using tritium in 2022. Largely heralded as a breakthrough for the future of nuclear energy, the facility and ICF tests also provide critical, experimental support for the SSMP. To better position the United States to capitalize on these long-term investments in science and technology, fusion energy leadership should not be ceded to other nations.
Plan of Action
Recommendation 1. Name a White House “Gigatons-to-Gigawatts” czar to coordinate a long-term tritium strategy and interagency cooperation harmonizing national security and fusion energy leadership goals.
A Senior Advisor on the National Security Team of the White House Office of Science and Technology Policy (OSTP) serving as the White House czar for GtG would (i) guide and lead efforts, (ii) coordinate interagency partners, and (iii) facilitate private/public stakeholder forums. Key interagency partners include:
- The Nuclear Weapons Council (NWC)
- DOE National Nuclear Security Administration (NNSA) Office of Tritium and Domestic Uranium Enrichment
- DOE NNSA Tritium Modernization Program (NA-19)
- DOE NNSA Office of Nuclear Material Integration (ONMI) (NA-532)
- DOE Office of Science
- The Office of Fusion Energy Sciences (FES) at DOE Office of Science
- DOE Advanced Research Projects Agency – Energy (ARPA-E)
- State Bureau of International Security and Nonproliferation (ISN)
- TVA Tritium Production Program
- Nuclear Regulatory Commission (NRC) Office of Nuclear Material Safety and Safeguards (NMSS)
- Savannah River National Lab (SRNL)
- Los Alamos National Lab (LANL)
- Pacific Northwest National Lab (PNNL)
- Idaho National Lab (INL) Safety and Tritium Applied Research (STAR)
- Environmental Protection Agency (EPA) Office of Radiation and Indoor Air (ORIA)
- Fusion Energy Sciences Advisory Committee (FESAC)
Key private partners include:
- Savannah River Nuclear Solutions (SRNS) and the Savannah River Tritium Enterprise (SRTE) program
- Westinghouse Government Services (WGS) Columbia Fuel Fabrication Facility (CFFF)
- Fusion Industry Association (FIA)
A central task of the GtG czar would be to coordinate with the NWC to review Presidential Policy Directive 9 (PPD-9) and associated/superseding planning documents related to the assessment of tritium demand requirements including (i) laboratory research, development, and surveillance and (ii) presidentially mandated tritium reserve. These two components of the tritium requirement could potentially be expanded to address GtG needs. If deemed appropriate, the President of the United States could be advised to expand the presidentially mandated reserve. Otherwise, the former requirement could be expanded based on optimal quantities to stand up a GtG program capability. A reference target would be the accumulation of ~10kg of tritium on projected timelines for commissioning full-scale FOAK fusion reactors.
The following recommendations could be coordinated by a GtG czar or done independently.
Recommendation 2. The Secretary of Energy should direct the Office of Science to evaluate the Milestone-Based Fusion Development Program for integrating GtG tritium production and supply targets with projected industry demands for commissioning fusion power plants.
The Milestone-Based Fusion Development Program has already provided awards of $46 million to 8 US companies. It is crucial to ensure that any tritium produced for a GtG program is not accumulated without a viable success path for FOAK fusion plant commissioning. Given the modest production capacities currently available at the WBN site, timelines of 5–10 years will be necessary to accumulate tritium. Each fuel cycle could allow for adjustments in production targets, but sufficient lead time will be required to anticipate and plan for necessary core changes and fuel-assembly production.
GtG tritium awards aligned with the Milestone-Based Fusion Development Program would also be more viable and attractive if costs were equitably shared between private awardees and the DOE. The U.S. Government produces tritium at WBN at a premium of ~$50,000/g whereas the market rate for tritium produced in Canada is closer to $30,000/g. A fusion company awarded tritium through the GtG program should be required to pay the prevailing market rate for tritium upon extraction at the Savannah River Site (SRS). This would allow a fusion company to benefit from increased tritium availability, while the DOE shoulders the cost differences of Tritium-Producing Burnable Absorber Rod (TPBAR) production methods. Additionally, this pay-as-you-go requirement will incentivize fusion energy companies to lay out realistic timeframes for FOAK reactor deployments.
The Director of the Office of Science should also direct the FESAC to prepare a report on tritium demand scenarios that would apply to leading fusion technology development timelines and assess the necessary tritium breeding efficiencies needed to sustain fusion power plant operations. The FESAC should give special consideration to projecting possible mitigation and recovery strategies for tritium breeding shortfalls. The committee should also provide thresholds for FOAK fusion reactors’ short-term recoverability from tritium breeding shortfalls. Tritium quantities based on this FESAC report should be considered for future tritium hedges after these fusion reactors begin power operations.
Recommendation 3. The NNSA ONMI (NA-532) should coordinate an interagency review of the tritium supply chain infrastructure.
Raising tritium production targets beyond previously projected requirements would necessitate review from TPBAR assembly at Westinghouse’s CFFF, irradiation at TVA’s Watts Bar Reactors, and then extraction and processing through the SRTE program at SRS. Because this review naturally involves civilian reactors and the transport of nuclear materials the NRC should also be consulted to ensure regulatory compliance is maintained. This review will provide realistic bounding limits to the quantities of tritium and production timelines that could be designated for a GtG program. The outcome of this review will inform industry-facing efforts to better assess how additional tritium supplies could best support fusion energy R&D and pilot plant commissioning.
As part of this process, the NA-532 office should determine which existing tritium supply chain models are best suited for assessing commercial applications, including the LANL Tritium Supply and Demand Model and those developed internally by the NNSA. If no model is determined fit for purpose, then a new model should be developed to best capture the dynamics of commercial fusion R&D. In any case, existing models should form the basis for integrating military requirements and civilian markets to ensure a GtG program adequately accounts for both.
An added-value option for this recommendation would be to prepare an unclassified and publicly accessible version of the commercial tritium supply chain model. This would reinforce the transparency and public accountability already built into the production of tritium in the commercial power reactors at Watts Bar. Furthermore, such a resource would also help explain the rationale and intent behind the use of public funds to support fusion R&D and the commissioning of FOAK fusion reactors.
Recommendation 4. The Secretary of Energy should direct a review of DOE Technical Standards for addressing tritium-related radiological risks.
While the general scientific consensus is that low-level tritium exposure poses negligible human health and ecosystem risks, there are several unknowns that should be better understood before the advent of fusion energy releases unprecedented quantities of tritium into the environment. This adequacy review should include at least [i] a comprehensive analysis of risks from Organically Bound Tritium (OBT) and [ii] more precisely quantifying and considering the potential for damaging mitochondrial DNA and fetuses. These efforts would help ensure the responsible, consent-based rollout of tritium-intensive technologies and allow for an informed public to better understand the magnitude of risks to be weighed against potential benefits.
Key DOE Technical Standards to include in this review:
- Derived Concentration Technical Standard (DOE-STD-1196-2022)
- Internal Dosimetry (DOE-STD-1121-2008 (Reaffirmed 2022))
- Nuclear Materials Control and Accountability (DOE-STD-1194-2019)
Recommendation 5. The Administrator of the Environmental Protection Agency (EPA) should direct the Office of Radiation and Indoor Air (ORIA) to assess the adequacy of radioactive dose calculations in the Federal Guidance Report on External Exposure to Radionuclides in Air, Water, and Soil (FGR 15) last issued in 2019.
This recommendation, along with recommendation 3 above, will provide sufficient lead time to address any uncertainties and unknowns regarding the radiological risks posed by tritium. As in this previous case, this adequacy review should include at least [i] a comprehensive analysis of risks from Organically Bound Tritium (OBT) and [ii] more precisely quantifying and considering the potential for damaging mitochondrial DNA and fetuses. FGR 15 currently calculates effective dose rates for “computational phantom” models of 6 different age groups, including newborns, that incorporate both male and female sex-specific tissues. However, effective dose rates and potential effects are not considered for developing fetuses. The uncertainty surrounding tritium’s radiological risks prompts an extensive precautionary approach to potential exposures for declared pregnant workers. However, the potential for higher levels of tritium exposure for pregnant members of the public should also be taken into consideration when assessing the radiological risks of fusion energy.
Conclusion
With a strategically calibrated GtG program, the United States could remain technology leaders in fusion energy and potentially reduce the rollout timeline of a multi-unit fleet by several years. In the context of state-level technological competition and a multi-polar nuclear security environment, years matter. A strategic GtG reserve will take years to plan and accumulate to ensure sufficient tritium is available at the right time.
The long-term utility of a GtG framework is not limited to the designation of new tritium production for peaceful use. Once nuclear-weapons states return to the negotiating table to reduce the number of nuclear weapons in the world, the United States would have a clear roadmap for repurposing the tritium from decommissioned weapons in support of fusion power. Previously, the United States held onto large reserves of this valuable and critical material for years while transitioning from military to civilian production. The years between 2025 and 2040 will provide more chances to put that material to productive use for fusion energy. Let us not waste this opportunity to ensure the U.S. remains at the vanguard of the fusion revolution.
This action-ready policy memo is part of Day One 2025 — our effort to bring forward bold policy ideas, grounded in science and evidence, that can tackle the country’s biggest challenges and bring us closer to the prosperous, equitable and safe future that we all hope for whoever takes office in 2025 and beyond.
PLEASE NOTE (February 2025): Since publication several government websites have been taken offline. We apologize for any broken links to once accessible public data.
A U.S. Government Accountability Office (GAO) report from 2000 provided unclassified approximations of total life-cycle cost ranged from ~$34,000 to $57,000 per gram of tritium. With several program delays and at least one major capital investment (i.e., a 500,000 gallon Tritiated Water Storage Tank (TWST) system) costing ~$20 million, the actual life-cycle costs are likely higher. The cost of tritium produced in Canada is closer to $30,000 per gram, but, as noted above, only fixed and limited amounts of tritium can be made available through this process.
This is unlikely. The SSMP projects tritium needs far enough into the future that demand changes could allow for adjustments to production levels over the span of 1–2 fuel cycles (i.e., one and a half to three years). Barring a catastrophic loss of military tritium reserves or a significant nuclear accident at Watts Bar, there is unlikely to be a tritium supply emergency requiring an immediate response.
Historical tritium production amounts and capacities at SRS remain restricted data. However, due to NRC regulatory requirements for commercial reactors, this information cannot be protected for tritium production at Watts Bar. Since tritium production transparency has been the norm since 2003, the United States may further demonstrate nuclear stockpile credibility by openly producing material in excess of current military requirements.
Unobligated fuel demand would slightly increase. Unobligated fuel requirements are largely a sunk cost. Regardless of how many TPBARs are being irradiated the entire core will be composed of unobligated fuel. However, increased tritium production (i.e., irradiating more TPBARs) would require additional fresh fuel bundles per fuel cycle. The 2024 SSMP currently projects meeting Watts Bar’s unobligated fuel needs through 2044.
This would possibly require new license amendments for each reactor, but if the amounts were below the previously analyzed conditions, then a new Environmental Impact Statement (EIS) would not be required. The current license for each reactor allows for the irradiation of up to 2,496 TPBARs per fuel cycle per reactor. The EIS analysis is bounded at a maximum of 6,000 TPBARs combined per fuel cycle. The average yield of each TPBAR is 0.95g of tritium.
Fusion industry leaders have demonstrated confidence that existing and future supplies of civilian-use tritium, while modest, are sufficient to fuel the necessary near-term R&D. In particular, the planned refurbishments to aging Canadian CANDU reactors and the additional delays at ITER have propped open the tritium window for several more years until tritium breeding blanket technologies can mature. However, tritium supply chain bottlenecks could constrain industry momentum and/or advantage states capable of backstopping any shortages.
New Nuclear Requires New Hiring at the NRC
The next generation of nuclear energy deployment depends on the Nuclear Regulatory Commission’s (NRC) willingness to use flexible hiring authorities to shape its workforce. Many analysts and policymakers propose increasing nuclear power production to ensure energy security and overall emissions reduction, and the U.S. recently joined 20 other countries in a pledge to triple global nuclear energy capacity by 2050. Additional nuclear deployment at this scale requires commercializing advanced reactor concepts or reducing capital costs for proven reactor technologies, and these outcomes rely on the capacity of the NRC to efficiently license and oversee a larger civilian nuclear industry. The ADVANCE Act, which became law in July, 2024, empowers the agency to accelerate licensing processes, mandates a new mission statement that reflects the benefits of nuclear energy, and provides additional direction to existing hiring flexibilities authorized by the Atomic Energy Act (AEA) of 1954. To meet expected demand for licensing and oversight, the NRC should not hesitate to implement new hiring practices under this direction.
The potential of the ADVANCE Act’s provisions should be understood in context of NRC’s existing authorities, practices, and history. NRC is exempt from the federal competitive hiring system for most positions. When Congress created the NRC in 1974 as a partial replacement of the Atomic Energy Commission (AEC), it maintained AEA provisions that allowed the AEC to hire without regard to civil service laws. Most NRC positions are in the Excepted Service, a category of positions across the federal workforce exempt from competitive hiring, which is particularly useful for highly-skilled positions that are impracticable to assess using traditional federal examining methods. The AEA allows NRC to hire staff to the Excepted Service provided salaries do not exceed grade 18 of the General Schedule (GS) (GS-16-18 were replaced with the Senior Executive Service in 1978) for scientific and technical positions and provided salaries for other positions follow the General Schedule when the occupation is comparable. Other agencies can hire to the Excepted Service in limited circumstances such as for candidates that are veterans or for specific occupations defined by the Office of Personnel Management (OPM).
Non-Competitive Hiring In Practice
Based on a review of NRC policies, procedures, and reports, NRC underuses its non-competitive hiring authorities provided under the AEA. Management Directives (or MDs, NRC’s internal policy documents) repeatedly state that NRC is exempt from competitive hiring under the AEA while outlining procedures that mirror government-wide practices derived from other laws and regulations such as the Senior Executive Service, Administrative Judges, experts and consultants, advisory committee members, and veterans, which are common flexible hiring pathways available to other agencies. MD 10.1 outlines NRC’s independent competitive merit system that generally follows OPM’s general schedule qualification standards. MD 10.13 on NRC’s non-competitive hiring practices under AEA authority is limited to part-time roles and student programs. While the policy includes a disclaimer that it covers only the most common uses, it does not include guidance on applying non-competitive hiring to other use cases.
The NRC has also been slow to reconcile its unique flexible hiring authorities with OPM Direct Hire Authority (DHA), a separate expedited process to hire to the Competitive Service. As far back as 2007, NRC hiring managers and human resources reported in Government Accountability Office interviews that DHA was highly desired and the agency was exploring how to obtain the authority. OPM denied NRC’s request for DHA the year before because it determined that it does not apply to NRC’s already-excepted positions under the AEA. NRC decided to replicate its own version of DHA that follows OPM’s restrictions for hiring of certain occupational categories. While this increased flexibility for hiring managers, a 2023 OIG audit found confusion among staff, managers, and directors about which laws and internal policies applied to DHA.
Making Sense of the ADVANCE Act
As NRC updates guidance on its version of DHA for hiring managers, the ADVANCE Act provides NRC with more direction for hiring to the Excepted Service. The law creates new categories of hires for positions that fill critical needs related to licensing, regulatory oversight, or matters related to NRC efficiency if the chair and the Executive Director for Operations (EDO) agree on the need. It specifies that the hires should be diverse in career level and have salaries commensurate with experience, with a maximum matching level III of the Executive Schedule. Additional limitations on the number of hires fall into two categories. The first category limits use of the authority to 210 hires at any time. The second category limits use of the authority to an additional 20 hires each fiscal year which are limited to a term of four years. The total number of staff serving at one time under the second category could reach 80 appointments if the authority is used to the maximum over four consecutive years. If NRC maximizes hiring in both categories each year for at least 4 years, the total number of staff serving at one time could reach 290, which is almost 7% of the current total NRC workforce. Several analyses and press releases mischaracterized or overlooked the specifics of these provisions, reporting the total number of 120 for the number of appointments in the first category, which could be a typo of 210 or a figure derived from a prior draft version of the bill. Appropriations are provided in NRC’s normal process of budget recovery through fees charged to license applicants.
The Regulatory Workforce for the Next Generation of Nuclear Power Plants
The capacity of the NRC to license new nuclear power plants and provide oversight to a larger number of operating reactors impacts the viability of nuclear power as part of the U.S.’s abundant and reliable energy system. For decades, the AEA has provided NRC staff with unique flexibility to shape a workforce to regulate the civilian nuclear energy and protect people and the environment. Under recent direction and specificity from Congress, the EDO should not hesitate to hire staff in new, specialized positions across the agency that are dedicated to implementing updates to licensing and oversight as mandated by the ADVANCE Act. In parallel, the EDO should work with the Office of Human Resources to promote NRC’s version of DHA to hiring managers more widely to solve long-standing hiring challenges for hard-to-recruit positions. Effective use of NRC’s broad hiring flexibilities are critical to realizing the next generation of nuclear energy deployment.
Restarting the Palisades Nuclear Plant and Keeping Momentum on Clean Energy
The Department of Energy (DOE) announced recently that it will finance the restart of a nuclear power plant through a new program to revitalize energy infrastructure and reduce greenhouse gas emissions. Restarting the Palisades Nuclear Power Plant, which was shut down in 2022, will be the first restarted nuclear power plant in U.S. history, bringing back much needed clean firm energy supply to Michigan, Illinois, and Indiana. DOE estimates that the addition of this clean capacity will prevent yearly emissions equivalent to that emitted by nearly one million gas-powered cars. The plant owners also shared intentions to use existing infrastructure to build two small modular reactors, a newer type of reactor technology that can be deployed more flexibly than existing commercial light-water reactors. DOE’s announcement is a significant step in addressing emerging energy needs and reducing emissions, but more is needed to ensure a successful plant restart and to expand clean energy capacity broadly.
Nuclear power was commercialized in the U.S. in the 1950s, and electricity generated by this technology accounts today for about 19% of the country’s electricity supply. Nuclear is a baseload power source, also called clean firm power, that complements generation from intermittent sources such as wind and solar energy. But in many cases, nuclear energy struggles to compete economically with other energy sources. The original decision to close the Palisades was primarily financial. Consumers Energy, the utility that purchased energy from the plant, intended to replace the nuclear energy with natural gas, which is ample and inexpensive. The dynamic is not unique—utilities are using more fossil fuels as the grid attempts to respond to a rapid increase in demand. But commercial light-water reactors, like those at the Palisades, are the most mature clean technology option to meet near-term energy needs while reducing emissions. The federal government should shape the market for nuclear power, or risk more plants shutting down—and making ambitious emissions reductions goals likely impossible to meet.
The conditional commitment from the DOE Loan Programs Office (LPO) to finance the Palisades restart ensures nuclear power is cost-competitive, and this particular type of loan is an important tool for DOE to develop and deploy more clean energy technologies. Since the loans are conditional on the companies meeting agreed-upon commitments, the arrangement allows DOE to closely monitor progress and halt funding if the project does not meet expectations. The LPO, established by Congress in 2005 to invest in critical energy and infrastructure projects, has found much success, especially with an increase in funding from the recent Inflation Reduction Act (IRA). Since the IRA passed in 2022, LPO has issued over $16 billion in conditional commitments and disbursed over $30 billion. The office’s approaches to lending seem to work well—for FY2023, they reported actual losses of only 3.1% of total funds disbursed. Other examples of recent conditional commitments include a real-time methane emissions monitoring network and a solar energy storage microgrid, reflecting investments across key clean energy technologies. But the Palisades commitment is unique as it is the first issued through DOE’s Energy Infrastructure Reinvestment program, which has $250 billion available to fund clean energy projects that revitalize or replace existing infrastructure. The $1.5 billion loan to Palisades will help fund refurbishment, upgrades, and testing to operate the plant for an estimated 25 years. Since the initial appropriations for this program expire in September of 2026, the DOE should act quickly to finance similar projects that revitalize existing infrastructure.
Outside of loans, the federal government can do more to support the restart and ensure other nuclear plants continue generating clean baseload energy for as long as safely possible. Next, the Nuclear Regulatory Commission (NRC) will need to amend the license of a plant it already classified in a state of decommissioning. The NRC formed the Palisades Restart Panel (PRP) to advise on the reviews required for this new regulatory situation. Although the primary objective of the PRP is to advise on the Palisades, NRC gave the panel the option to provide general recommendations if other licensees pursue a restart. Twenty other nuclear power reactor sites are in decommissioning status. To provide clarity to the nuclear industry on options for these sites, the panel should take advantage of this opportunity to advise generally on a process for restarts. The DOE should also signal whether it intends to make further investments in this area. This first-of-kind project could demonstrate that restarting plants is a fast and economical way to increase clean firm generating capacity.
Federal policymakers, agencies, and the private sector should consider additional options for expanding nuclear capacity at this moment when nuclear power is viewed favorably by most of the public and partisan division is low. For example, utilities could form consortiums to build multiple reactors of the same design, reducing risk and cost with the construction of each new reactor. The DOE could mass-acquire NRC permits on behalf of developers, or use the Foundation for Energy Security and Innovation (FESI) to accelerate licensing through stakeholder and community engagement. Congress could also consider categorical exclusions under the National Environmental Policy Act for actions that use existing energy infrastructure and have a net positive benefit to the environment, such as building nuclear power plants on former coal plant sites. The LPO has nearly $412 billion in loan authority to advance clean energy. It should continue to negotiate and award conditional commitments for more clean energy projects across the country, working closely with applicants and recipients to ensure adequate progress and effective use of taxpayer dollars. Other federal policymakers should keep momentum on DOE’s commitment to Palisades with further actions to keep nuclear power on the grid.
Engaging Coal Communities in Decarbonization Through Nuclear Energy
The United States is committed to the ambitious goal of reaching net-zero emissions globally by 2050, requiring rapid deployment of clean energy domestically and across the world. Reducing emissions while meeting energy demand requires firm power sources that produce energy at any time and in adverse weather conditions, unlike solar or wind energy. Advanced nuclear reactors, the newest generation of nuclear power plants, are firm energy sources that offer potential increases in efficiency and safety compared to traditional nuclear plants. Adding more nuclear power plants will help the United States meet energy demand while reducing emissions. Further, building advanced nuclear plants on the sites of former coal plants could create benefits for struggling coal communities and result in significant cost savings for project developers. Realizing these benefits for our environment, coal communities, and utilities requires coordinating and expanding existing efforts. The Foundation for Energy Security and Innovation (FESI), the US Department of Energy (DOE), and Congress should each take actions to align and strengthen advanced nuclear initiatives and engagement with coal communities in the project development process.
Challenge and Opportunity
Reducing carbon emissions while meeting energy demand will require the continued use of firm power sources. Coal power, once a major source of firm energy for the United States, has declined since 2009, due to federal and state commitments to clean energy and competition with other clean energy sources. Power generated from coal plants is expected to drop to half of current levels by 2050 as upwards of 100 plants retire. The DOE found that sites of retiring coal plants are promising candidates for advanced nuclear plants, considering the similarities in site requirements, the ability to reuse existing infrastructure, and the overlap in workforce needs. Advanced nuclear reactors are the next generation of nuclear technology that includes both small modular reactors (SMRs), which function similar to traditional light-water reactors except on a smaller site, and non-light-water reactors, which are also physically smaller but use different methods to control reactor temperature. However, the DOE’s study and additional analysis from the Bipartisan Policy Center also identified significant challenges to constructing new nuclear power plants, including the risk of cost overrun, licensing timeline uncertainties, and opposition from communities around plant sites. Congress took steps to promote advanced nuclear power in the Inflation Reduction Act and the CHIPS and Science Act, but more coordination is needed. To commercialize advanced nuclear to support our decarbonization goals, the DOE estimates that utilities must commit to deploying at least five advanced nuclear reactors of the same design by 2025. There are currently no agreements to do so.
The Case for Coal to Nuclear
Coal-dependent communities and the estimated 37,000 people working in coal power plants could benefit from the construction of advanced nuclear reactors. Benefits include the potential addition of more than 650 jobs, about 15% higher pay on average, and the ability for some of the existing workforce to transition without additional experience, training, or certification. Jobs in nuclear energy also experience fewer fatal accidents, minor injuries, and harmful exposures than jobs in coal plants. Advanced nuclear energy could revitalize coal communities, which have suffered labor shocks and population decline since the 1980s. By embracing advanced nuclear power, these communities can reap economic benefits and create a pathway toward a sustainable and prosperous future. For instance, in one case study by the DOE, replacing a 924 MWe coal plant with nuclear increased regional economic activity by $275 million. Before benefits are realized, project developers must partner with local communities and other stakeholders to align interests and gain public support so that they may secure agreements for coal-to-nuclear transition projects.
Communities living near existing nuclear plants tend to view nuclear power more favorably than those who do not, but gaining acceptance to construct new plants in communities less familiar with nuclear energy is challenging. Past efforts using a top-down approach were met with resistance and created a legacy of mistrust between communities and the nuclear industry. Stakeholders can slow or stop nuclear construction through lawsuits and lengthy studies under the National Environmental Policy Act (NEPA), and 12 states have restrictions or total bans on new nuclear construction. Absent changes to the licensing and regulatory process, project developers must mitigate this risk through a process of meaningful stakeholder and community engagement. A just transition from coal to nuclear energy production requires developers to listen and respond to local communities’ concerns and needs through the process of planning, siting, licensing, design, construction, and eventual decommissioning. Project developers need guidance and collective learning to update the siting process with more earnest practices of engagement with the public and stakeholders. Coal communities also need support in transitioning a workforce for nuclear reactor operations.
Strengthen and Align Existing Efforts
Nuclear energy companies, utilities, the DOE, and researchers are already exploring community engagement and considering labor transitions for advanced nuclear power plants. NuScale Power, TerraPower, and X-energy are leading in both the technical development of advanced nuclear and in considerations of community benefits and stakeholder management. The Utah Associated Municipal Power Systems (UAMPS), which is hosting NuScale’s demonstration SMR, spent decades engaging with communities across 49 utilities over seven states before signing an agreement with NuScale. Their carbon-free power project involved over 200 public meetings, resulting in several member utilities choosing to pursue SMRs. Universities are collaborating with the Idaho National Laboratory to analyze energy markets using a multidisciplinary framework that considers community values, resources, capabilities, and infrastructure. Coordinated efforts by researchers near the TerraPower Natrium demonstration site investigate how local communities view the cost, benefits, procedures, and justice elements of the project.
The DOE also works to improve stakeholder and community engagement across multiple offices and initiatives. Most notably, the Office of Nuclear Energy is using a consent-based siting process, developed with extensive public input, to select sites for interim storage and disposal of spent nuclear fuel. The office distributed $26 million to universities, nonprofits, and private partners to facilitate engagement with communities considering the costs and benefits of hosting a spent fuel site. DOE requires all recipients of funds from the Infrastructure Investment and Jobs Act and the Inflation Reduction Act, including companies hosting advanced nuclear demonstration projects, to submit community benefits plans outlining community and labor organization engagement. The DOE’s new Commercial Liftoff Reports for advanced nuclear and other clean energy technologies are detailed and actionable policy documents strengthened by the inclusion of critical societal considerations.
Through the CHIPS and Science Act, Congress established or expanded DOE programs that promote both the development of advanced nuclear on sites of former coal plants and the research of public engagement for nuclear energy. The Nuclear Energy University Program (NEUP) has funded technical nuclear energy research at universities since 2009. The CHIPS Act expanded the program to include research that supports community engagement, participation, and confidence in nuclear energy. The Act also established, but did not fund, a new advanced nuclear technology development program that prioritizes projects at sites of retiring coal plants and those that include elements of workforce development. An expansion of an existing nuclear energy training program was cut from the final CHIPS Act, but the expansion is proposed again in the Nuclear Fuel Security Act of 2023.
More coordination is required among DOE, the nuclear industry, and utilities. Congress should also take action to fund initiatives authorized by recent legislation that enable the coal-to-nuclear transition.
Plan of Action
Recommendations for Federal Agencies
Recommendation 1. A sizable coordinating body, such as the Foundation for Energy Security and Innovation (FESI) or the Appalachian Regional Commission (ARC), should support the project developer’s efforts to include community engagement in the siting, planning, design, and construction process of advanced nuclear power plants.
FESI is a new foundation to help the DOE commercialize energy technology by supporting and coordinating stakeholder groups. ARC is a partnership between the federal government and Appalachian states that supports economic development through grantmaking and conducting research on issues related to the region’s challenges. FESI and ARC are coordinating bodies that can connect disparate efforts by developers, academic experts, and the DOE through various enabling and connecting initiatives. Efforts should leverage existing resources on consent-based siting processes developed by the DOE. While these processes are specific to siting spent nuclear fuel storage facilities, the roadmap and sequencing elements can be replicated for other goals. Stage 1 of the DOE’s planning and capacity-building process focuses on building relationships with communities and stakeholders and engaging in mutual learning about the topic. FESI or ARC can establish programs and activities to support planning and capacity building by utilities and the nuclear industry.
FESI could pursue activities such as:
- Hosting a community of practice for public engagement staff at utilities and nuclear energy companies, experts in public engagement methods design, and the Department of Energy
- Conducting activities such as stakeholder analysis, community interest surveys, and engagement to determine community needs and concerns, across all coal communities
- Providing technical assistance on community engagement methods and strategies to utilities and nuclear energy companies
ARC could conduct studies such as stakeholder analysis and community interest surveys to determine community needs and concerns across Appalachian coal communities.
Recommendation 2. The DOE should continue expanding the Nuclear Energy University Program (NEUP) to fund programs that support nontechnical nuclear research in the social sciences or law that can support community engagement, participation, and confidence in nuclear energy systems, including the navigation of the licensing required for advanced reactor deployment.
Evolving processes to include effective community engagement will require new knowledge in the social sciences and shifting the culture of nuclear education and training. Since 2009, the DOE Office of Nuclear Energy has supported nuclear energy research and equipment upgrades at U.S. colleges and universities through the NEUP. Except for a few recent examples, including the University of Wyoming project cited above, most projects funded were scientific or technical. Congress recognized the importance of supporting research in nontechnical areas by authorizing the expansion of NEUP to include nontechnical nuclear research in the CHIPS and Science Act. DOE should not wait for additional appropriations to expand this program. Further, NEUP should encourage awardees to participate in communities of practice hosted by FESI or other bodies.
Recommendation 3. The DOE Office of Energy Jobs and the Department of Labor (DOL) should collaborate on the creation and dissemination of training standards focused on the nuclear plant jobs for which extensive training, licensing, or experience is required for former coal plant workers.
Sites of former coal plants are promising candidates for advanced nuclear reactors because most job roles are directly transferable. However, an estimated 23% of nuclear plant jobs—operators, senior managers, and some technicians—require extensive licensing from the Nuclear Regulatory Commission (NRC) and direct experience in nuclear roles. It is possible that an experienced coal plant operator and an entry-level nuclear hire would require the same training path to become an NRC-licensed nuclear plant operator.
Supporting the clean energy workforce transition fits within existing priorities for the DOE’s Office of Energy Jobs and the DOL, as expressed in the memorandum of understanding signed on June 21, 2022. Section V.C. asserts the departments share joint responsibility for “supporting the creation and expansion of high-quality and equitable workforce development programs that connect new, incumbent, and displaced workers with quality energy infrastructure and supply chain jobs.” Job transition pathways and specific training needs will become apparent through additional studies by interested parties and lessons from programs such as the Advanced Reactor Demonstration Program and the Clean Energy Demonstration Program on Current and Former Mine Land. The departments should capture and synthesize this knowledge into standards from which industry and utilities can design targeted job transition programs.
Recommendations for Congress
Recommendation 4. Congress should fully appropriate key provisions of the CHIPS and Science Act to support coal communities’ transition to nuclear energy.
- Appropriate $800 million over FY2024 to FY2027 to establish the DOE Advanced Nuclear Technologies Federal Research, Development, and Demonstration Program: The CHIPS and Science Act established this program to promote the development of advanced nuclear reactors and prioritizes projects at sites of retiring coal power plants and those that include workforce development programs. These critical workforce training programs need direct funding.
- Appropriate an additional $15 million from FY2024 to FY2025 to the NEUP: The CHIPS and Science Act authorizes an additional $15 million from FY 2023 to FY 2025 to the NEUP within the Office of Nuclear Energy, increasing the annual total amount from $30 million to $45 million. Since CHIPS included an authorization to expand the program to include nontechnical nuclear research, the expansion should come with increased funding.
Recommendation 5. Congress should expand the Nuclear Energy Graduate Traineeship Subprogram to include workforce development through community colleges, trade schools, apprenticeships, and pre-apprenticeships.
The current Traineeship Subprogram supports workforce development and advanced training through universities only. Expanding this direct funding for job training through community colleges, trade schools, and apprenticeships will support utilities’ and industries’ efforts to transition the coal workforce into advanced nuclear jobs.
Recommendation 6. Congress should amend Section 45U, the Nuclear Production Tax Credit for existing nuclear plants, to require apprenticeship requirements similar to those for future advanced nuclear plants covered under Section 45Y, the Clean Energy Production Tax Credit.
Starting in 2025, new nuclear power plant projects will be eligible for the New Clean Energy Production and Investment Tax Credits if they meet certain apprenticeship requirements. However, plants established before 2025 will not be eligible for these incentives. Congress should add apprenticeship requirements to the Nuclear Production Tax Credit so that activities at existing plants strengthen the total nuclear workforce. Credits should be awarded with priority to companies implementing apprenticeship programs designed for former coal industry workers.
Conclusion
The ambitious goal of reaching net-zero emissions globally requires the rapid deployment of clean energy technologies, in particular firm clean energy such as advanced nuclear power. Since the 1980s, communities around coal power plants have suffered from industry shifts and will continue to accumulate disadvantages without support. Coal-to-nuclear transition projects advance the nation’s decarbonization efforts while creating benefits for developers and revitalizing coal communities. Utilities, the nuclear industry, the DOE, and researchers are advancing community engagement practices and methods, but more effort is required to share best practices and ensure coordination in these emerging practices. FESI or other large coordinating bodies should fill this gap by hosting communities of practice, producing knowledge on community values and attitudes, or providing technical assistance. DOE should continue to promote community engagement research and help articulate workforce development needs. Congress should fully fund initiatives authorized by recent legislation to promote the coal to nuclear transition. Action now will ensure that our clean firm power needs are met and that coal communities benefit from the clean energy transition.
Transitioning coal miners directly into clean energy is challenging considering the difference in skills and labor demand between the sectors. Most attempts to transition coal miners should focus on training in fields with similar skill requirements, such as job training for manufacturing roles within the Appalachian Climate Technology Coalition. Congress could also provide funding for unemployed coal miners to pursue education for other employment.
A significant challenge is aligning the construction of advanced nuclear plants with the decommissioning of coal plants. Advanced nuclear project timelines are subject to various delays and uncertainties. For example, the first commercial demonstration of small modular reactor technology in the United States, the TerraPower plant in Wyoming, is delayed due to the high-assay low-enriched uranium supply chain. The Nuclear Regulatory Commission’s licensing process also creates uncertainty and extends project timelines.
Methods exist to safely contain radioactive material as it decays to more stable isotopes. The waste is stored on site at the power plant in secure pools in the shorter term and in storage casks capable of containing the material for at least 100 years in the longer term. The DOE must continue pursuing interim consolidated storage solutions as well as a permanent geological repository, but the lack of these facilities should not pose a significant barrier to constructing advanced nuclear power plants. The United States should also continue to pursue recycling spent fuel.
More analysis is required to better understand these impacts. A study conducted by Argonne National Laboratory found that while the attributes of spent fuel vary by the exact design of reactor, overall there are no unique challenges to managing fuel from advanced reactors compared to fuel from traditional reactors. A separate study found that spent fuel from advanced reactors will contain more fissile nuclides, which makes waste management more challenging. As the DOE continues to identify interim and permanent storage sites through a consent-based process, utilities and public engagement efforts must interrogate the unique waste management challenges when evaluating particular advanced nuclear technology options.
Similar to waste output, the risk of proliferation from advanced reactors varies on the specific technologies and requires more interrogation. Some advanced reactor designs, such as the TerraPower Natrium reactor, require the use of fuel that is more enriched than the fuel used in traditional designs. However, the safeguards required between the two types of fuel are not significantly different. Other designs, such as the TerraPower TWR, are expected to be able to use depleted or natural uranium sources, and the NuScale VOYGR models use traditional fuel. All reactors have the capacity to produce fissile material, so as the United States expands its nuclear energy capabilities, efforts should be made to expand current safeguards limiting proliferation to fuel as it is prepared for plants and after it has been used.
Solutions for mitigating climate change, advances in nuclear energy, and US leadership in high-performance computing discussed in two key House Science Committee hearings
Climate solutions and nuclear energy
The full House Science, Space, and Technology Committee discussed climate hurdles and solutions in a January 15 hearing titled, “An update on the climate crisis: From science to solutions.” Interestingly, the main point of debate during this hearing was not whether climate change was occurring, but rather the economic impacts of climate change mitigation. As predicted, the debate was split down party lines.
While the Democrats emphasized the negative consequences of climate change and the need to act, several Republican members insisted that China and India rein in their greenhouse gas emissions first.
Congressman Mo Brooks (R, AL-05) asked the most heated series of questions during the hearing, related to India and China’s carbon emissions. He asked if there was a way to force both to reduce their emissions, which, according to a report by the European Union, have seen increases of 305% and 354%, respectively, between 1990 and 2017.
Democrats focused their questions to highlight the science behind climate change. Chairwoman Eddie Bernice Johnson (D, TX-30) asked each witness about the biggest hurdles in their fields. Richard Murray, Deputy Director and Vice President for Research at Woods Hole Oceanographic Institution, said that more investments in large-scale ocean observations and data are needed. Pamela McElwee, Associate Professor of Human Ecology at Rutgers, said that a lot of advances in land conservation can be made with existing technology, but that investments in genetic modification of crops to restore nutrients to the soil, for example, could be developed. Heidi Steltzer, Professor of Environment and Sustainability at Fort Lewis College, encouraged the inclusion of diverse perspectives in climate research to develop the most creative solutions. Congressman Paul Tonko (D, NY-20) summed up the Democrats’ views on climate change by stating that the climate science performed by researchers like the witnesses should inform federal action and that inaction on this issue is costly.
While committee Republicans expressed concerns over the impact of climate regulations on business, members of the committee did emphasize the importance of renewing U.S. leadership in nuclear power, pointing to competition from Russia and China. Nuclear power continues to be the largest source of carbon free electricity in the country.
One of the witnesses, Michael Shellenberger, Founder and President of Environmental Progress noted that the US’ ability to compete internationally in nuclear energy was declining as Russia and China rush to complete new power plants. Losing ground in this area, he added, negatively impacts the U.S.’ reputation as a developer of cutting edge energy technology and dissuades developing countries interested in building nuclear power plants from contracting with the U.S.
As the impacts of climate change take their toll in California, the Caribbean, Australia, and elsewhere, the U.S. Congress remains divided on how to address it.
We thank our community of experts for helping us create an informative resource and questions for the committee.
Supercomputing a high priority for DOE Office of Science
While last week’s House Science Subcommittee on Energy hearing about research supported by the Department of Energy (DOE) Office of Science touched on a range of issues, competition with China on high-performance computing took center stage.
The big milestone that world powers are competing to reach in the high-performance computing field is the development of the first-ever exascale computer. An exascale computer would greatly enhance research areas like materials development for next-generation batteries, seismic analysis, weather and climate modeling, and even clinical health studies like “identifying risk factors for suicide and best practices for intervention.” It would be about a million times faster than a consumer desktop computer, operating at a quintillion calculations per second. The U.S., China, Japan, and European Union are all working to complete the first exascale system.
In the competition to develop faster and faster supercomputers, China has made rapid progress. In 2001, none of the 500 fastest supercomputers were made in China. As of June 2019, 219 of the 500 fastest supercomputers had been developed by China, and the US had 116. Notably, when the computational power of all these systems is totaled up for each country, China controls 30 percent of the world’s high-performance computing resources, while the U.S. controls 38 percent. In the past, China had asserted that it would complete an exascale computing system this year; however, it is unclear if the country will meet its goal.
A U.S. exascale system due in 2021 – Aurora – is being built at Argonne National Lab in Illinois, and hopes are high that it will be the world’s first completed exascale computer. During the hearing, Representatives Dan Lipinski (D, IL-03) and Bill Foster (D, IL-11) both raised the issue of progress on the project. According to DOE Office of Science director Dr. Christopher Fall, the Aurora project is meeting its benchmarks, with headway being made not only on the hardware, but also on a “once-in-a-generation” reworking and modernization of the software stack that will run on the system, as well as developing high-speed internet for linking generated data with the computation of that data. DOE believes that the U.S. is in a strong position to complete the first-ever exascale computing system, and that our holistic approach to high-performance computing is something that is missing from competitors’ strategies, giving the U.S. even more of an edge.
In addition to the Aurora project, two more exascale computing projects are underway at U.S. National Labs. Frontier, at Oak Ridge National Laboratory in Tennessee, is also projected to deploy in 2021, while El Capitan, based at Lawrence Livermore National Laboratory in California, should launch in 2022. El Capitan will only be used by individuals in the national security field.
In addition to research in high-performance computing, the diverse and impactful science supported by the DOE Office of Science is truly something to protect and promote. To review the full hearing, click here.
Suggestions about Japan’s Nuclear Fuel Recycling Policy Based on U.S. Concerns
To date, Japan’s peaceful nuclear energy use has taken the form of a nuclear fuel recycling policy that reprocesses spent fuel and effectively utilizes the plutonium retrieved in light water reactors (LWRs) and fast reactors (FRs). With the aim to complete recycling domestically, Japan has introduced key technology from abroad and has further developed its own technology and industry. However, Japan presently seems to have issues regarding its recycling policy and plutonium management.
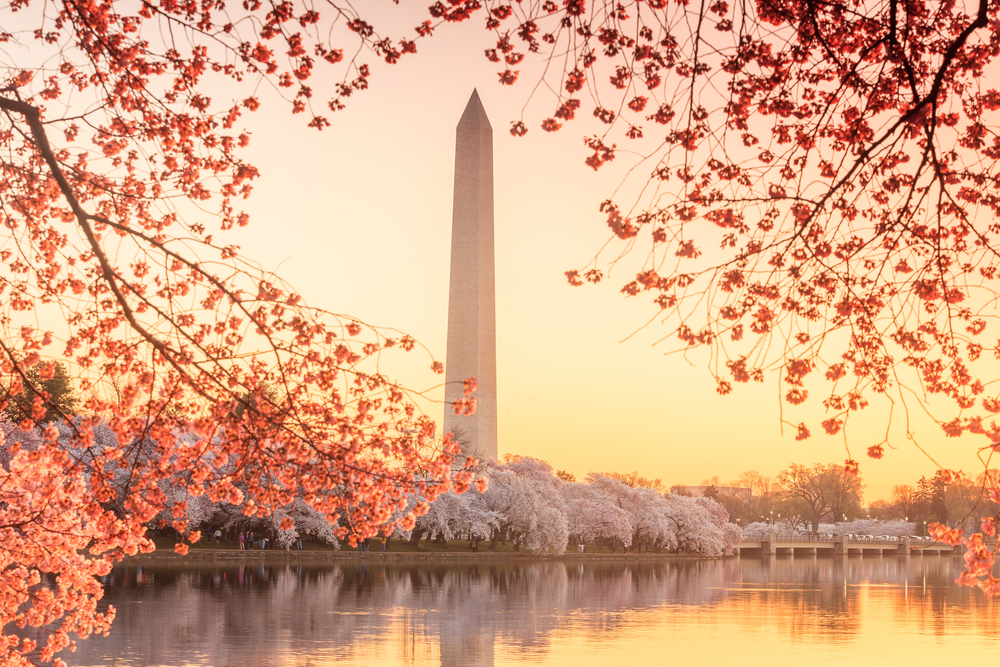
Because of recent increasing risks of terrorism and nuclear proliferation in the world, the international community seeks much more secure use of nuclear energy. All of the countries that store plutonium (which can be used for making nuclear weapons) must make the best efforts possible to decrease it. Taking this into account, concerns about Japan’s problem of plutonium management have been growing in the international community and Japan’s accountability for its recycling policy is essential.
In this paper, Yusei Nagata, an FAS Research Fellow from MEXT, Japan, analyzes U.S. experts’ opinions and concerns about Japan’s problem and considers what Japan can (and should) do to solve it.
Read the full report here.
Moving Advanced Nuclear Energy Systems to Global Deployment
In the FAS Special Report entitled, Moving Advanced Nuclear Energy Systems to Global Deployment, Charles D. Ferguson, FAS President, identifies the major factors that will affect deployment of advanced reactors (often referred to as Generation IV reactors) in the coming years to decades and analyzes what industry and governments need to do to move forward toward the ultimate goal of widespread deployment of potentially hundreds of highly energy-efficient, much safer, more proliferation-resistant, and economically-competitive nuclear power systems. Moreover, the report looks at lessons learned from the history of development and deployment of Generation II and III reactors and seeks to learn explicitly about the reasons for the predominant use of light water reactors. It then seeks to apply these lessons to current efforts to develop advanced nuclear energy systems. In the process of that assessment, the report reviews the status of the global cooperative and national efforts to develop and eventually deploy advanced nuclear energy systems. The main intentions of the report are to provide a guide to policymakers in the form of findings that lay out potential pathways to forward deployment of one or more advanced nuclear power systems within the next ten to twenty years.
A full PDF version of the report can be found here.
Look to Texas Rather Than Nevada for a Site Selection Process on Nuclear Waste Disposal
Republican gains in the 2014 midterm elections have refocused attention on a number of policy areas–including nuclear waste storage. Although President Obama has consistently championed nuclear power by providing federal loan guarantees for new reactors and placing nuclear power among the “clean energy” sources targeted for an 80 percent share of the nation’s electricity production by 2035, he has also placed the viability of nuclear power in doubt by thwarting efforts to build a high level radioactive waste repository at Yucca Mountain, Nevada. Several newspapers around the country have run editorials arguing that the Yucca Mountain ought to be revived or even, as the Chicago Tribune suggested, “fast-tracked.” Arguments like these emphasize the risks associated with our current interim storage of spent fuel at more than one hundred power plants in close proximity to population centers throughout the country, commitments for disposal capacity the federal government owes utilities and contaminated legacy sites like those in South Carolina and Washington State, and the amount of research and spending that has already been devoted to investigating the suitability of the Yucca Mountain site.
However, it is unlikely that Yucca Mountain will ever receive shipments of nuclear waste. Nevada’s persistent and successful efforts to thwart the Yucca Mountain project and the Nuclear Waste Policy Act of 1982 are likely to continue as they demonstrate the futility of a policy that forces disposal on an unwilling host state. Three years ago the Blue Ribbon Commission on America’s Nuclear Future said as much, recommending instead a “consent-based” approach to siting nuclear waste storage and disposal facilities. How would such an approach work?
For the past three years, Texas has been accepting what so many other states and localities have rejected in past decades- radioactive waste from the nation’s nuclear power plants. A newly opened private facility operated by Waste Control Specialists in Andrews County, Texas has been receiving shipments of low-level radioactive waste from multiple states. This year, the Texas Commission on Environmental Quality has amended the license for the Andrews County site to more than triple its capacity and it can begin accepting “Greater Than Class C Waste”- the most highly radioactive materials in the low-level radioactive waste stream, as well as depleted uranium. Residents and elected officials in Andrews County are now considering whether or not to support a proposal for a high-level radioactive waste disposal facility.
We should take a closer look at past developments in Nevada and more recent decisions in Texas to guide our future nuclear waste policy. These two states are engaging with different aspects of the nuclear waste stream, governed by very different policy approaches. Nevada’s efforts to thwart the Yucca Mountain project are rooted in the coercive approach codified in the Nuclear Waste Policy Act of 1982. In contrast, the willingness of Texas to establish new disposal capacity stems from the Low-level Radioactive Waste Policy Act of 1980—a law that expanded the authority of states hosting disposal sites in an effort to overcome state opposition to waste sites in the midst of an urgent shortage of disposal capacity.
First, let’s consider the troublesome politics that has infused the Nevada case. The Nuclear Waste Policy Act of 1982 established a scientific site selection process for an eastern and western waste repository. However, President Reagan abandoned this process in 1986 by halting the search for an eastern site amid fears of midterm election losses in potential host states of Wisconsin, Georgia and North Carolina. In 1987, Congress abandoned the search for a western site when House Speaker Jim Wright (D-TX), and House Majority Leader Tom Foley (D-WA), amended the law to remove Texas and Washington from consideration. The amended law became known as the “Screw Nevada” plan because it designated Yucca Mountain as the sole site for the waste repository.
While politics effectively trumped science in the selection of Yucca Mountain, opponents- led by Senator Harry Reid of Nevada- have employed politics to effectively thwart the project. In 2005, Reid placed 175 holds on President Bush’s nominations for various executive appointments until Bush finally nominated Reid’s own science advisor, Gregory Jaczko, to the Nuclear Regulatory Commission (NRC). In 2006 Reid persuaded the Democratic National Committee to move the Nevada caucuses to the front of the 2008 presidential primary calendar, prompting each candidate to oppose Yucca Mountain. President Obama fulfilled his campaign promise by tapping Jaczko to chair the NRC and dismantling Yucca Mountain. Each year the President’s budget proposals zeroed out funding for the facility, the NRC defunded the license review process and the Department of Energy has continued to mothball the project. Although court decisions have forced the administration to begin reviewing the project, progress has been slow and in the meantime the Yucca facility offices have been shuttered, workforce eliminated, and computers, equipment and vehicles have been surplused. Jaczko was forced to resign amidst concern from other NRC members that his management style thwarted decision making processes. However, Jaczko’s chief counsel, Stephen Burns was sworn in as the commissioner of the NRC on November 5, 2014.
We should expect, accept, and plan for such political maneuvering. Our system of locally accountable representatives empowers individual office holders with a wealth of substantive and procedural tools that make all nuclear politics local. Any decision making on this issue will be a political contest to locate or avoid the waste. Consequently, if there is to be a politically feasible nuclear waste repository, it will require a willing host. Money and the promise of jobs alone have not proven alluring enough for acceptance of such a project. We would do better to embrace our decentralized politics and offer the host significant authority over the waste stream.
This is the current situation that Texas enjoys: Congress gave states responsibility for establishing low-level radioactive waste sites and, as an incentive, enabled states to join interstate compacts. Once approved by Congress, a compact has the authority to accept or decline waste imports from other states, which is a power that is normally not extended to states because it violates the interstate commerce clause of the U.S. Constitution. Texas is in a compact with Vermont, and as host state, Texas shapes the waste market by determining disposal availability for other states. Texas also has authority to set fees, taxes, and regulations for disposal in collaboration with federal agencies. Compacts can dissolve and host states can cease accepting waste altogether at a future date. While even under these provisions most states will refuse to host radioactive waste, the extension of state authority at least courts the possibility (as in Texas) of the rare case that combines an enthusiastic local host community in a relatively suitable location, a supportive state government, and a lack of opposition from neighboring communities and states. This approach better meets our democratic expectations because it confronts the local, state and national politics openly and directly, courting agreement at each level and extending authority over the waste stream to the unit of government bearing responsibility for long term disposal within its borders.
What if we adopt this approach and there is no willing host for spent fuel at a technically suitable site? What if a site is established, but at some future date the host state and compact exercise authority refuse importation or dissolve altogether? We would be left with interim onsite storage- the same result our current predictably failed policy approach has left us in. If there is no willing host, or if long term disposal is less certain due to the host’s authority over the waste stream, we also gain authentic and valuable feedback on societal support for nuclear energy. That is, our willingness to provide for waste disposal in a process compatible with our democratic norms and decentralized political system should influence our decisions on nuclear energy production and waste generation.
Nuclear Power and Nanomaterials: Big Potential for Small Particles
Nuclear power plants are large, complex, and expensive facilities. They provide approximately 19 percent of U.S. electricity power supply,1 and in the process consume enormous quantities of water. However, a class of very small particles may be gearing up to lend a helping hand in making power plants more efficient and less costly to operate. This article will briefly introduce nanomaterials and discuss ways in which some of these particles may make nuclear power plants more efficient.
The race to synthesize, engineer, test, and apply new nanoscale materials for solving difficult problems in energy and defense is in full swing. The past twenty five years have ushered in an era of nanomaterials and nanoparticles – objects with at least one dimension between 1 and 100 nanometers2 – and researchers are now implementing these materials in areas as disparate as neuroscience and environmental remediation. To provide a sense of scale, most viruses are a few hundred nanometers in size, most bacteria are a few thousand nanometers in size, and a period at the end of a sentence is about a million nanometers. This new category of materials has ignited the imaginations of scientists and engineers who envision nanomaterials capable of tackling difficult problems in energy, healthcare, and electronics.
Nanomaterials are not new, and indeed occur naturally all over Earth. This includes viruses, the coatings of a lotus leaf, the bottom of a gecko’s foot, and some finely powdered clays. These objects represent natural materials with significant, and often highly functional, nanoscale features. Some researchers have even discovered signs of nanoscale materials in space.3 One of the oldest documented applications of nanomaterials dates back to the Lycurgus Cup, a 4th century Roman glass which was made out of a glass containing gold and silver particles. The result is a glass that appears green when lit from the outside, but red when lit from the inside.4 The effect results from the glass filtering various wavelengths of light differently depending on the various lighting conditions. Of course, the Romans did not know they were using nanoparticles in the process of making this glass.
But what makes nanoparticles interesting or unique? The answer to this question depends on the specific material and application, but a few themes persist. Because of their small size, the physical principles governing how particles behave and interact with their environments change. Some of these changes are due to how basic properties such as volume and surface area change as an object becomes smaller. As a sphere shrinks, the ratio of the surface area to the volume grows. This has far reaching implications for how particles interact with light, heat, and other particles. Visionary researchers are now looking into ways in which these interesting properties may make nuclear power plants more efficient.
One important implication for our discussion is the flow of thermal energy. Consider the process of transferring the thermal energy of your body from your hands to an ice cube. Clearly, you are (hopefully!) warmer than the ice cube. If you place the ice cube on a chilled dish and touch the ice cube with one finger, the cube will melt, but probably fairly slowly. Placing your entire hand over the top half of the ice cube increases the melting rate, and placing the ice cube in your hand and closing your fist further increases the melting rate. This is an example of thermal energy transfer via conduction. Conductive heat transfer from one object to another depends on the area over which the thermal transfer takes place. A larger contact surface area leads to faster conduction. But how does this relate to nanoparticles? As a particle becomes very small, the ratio of the particle’s surface area to its volume increases very rapidly. Since thermal conduction through volume is a function of surface area, particles with large ratios of surface area to volume are able to change temperature very quickly. If you place a large quantity of small cold particles in a warm body of water, the particles will heat quickly. If you take the same volume of particles, but instead compress it into one large particle, then that large particle will warm slowly. As this surface area to volume ratio increases with decreasing size, a general trend is for smaller particles to transfer heat more effectively than larger particles.
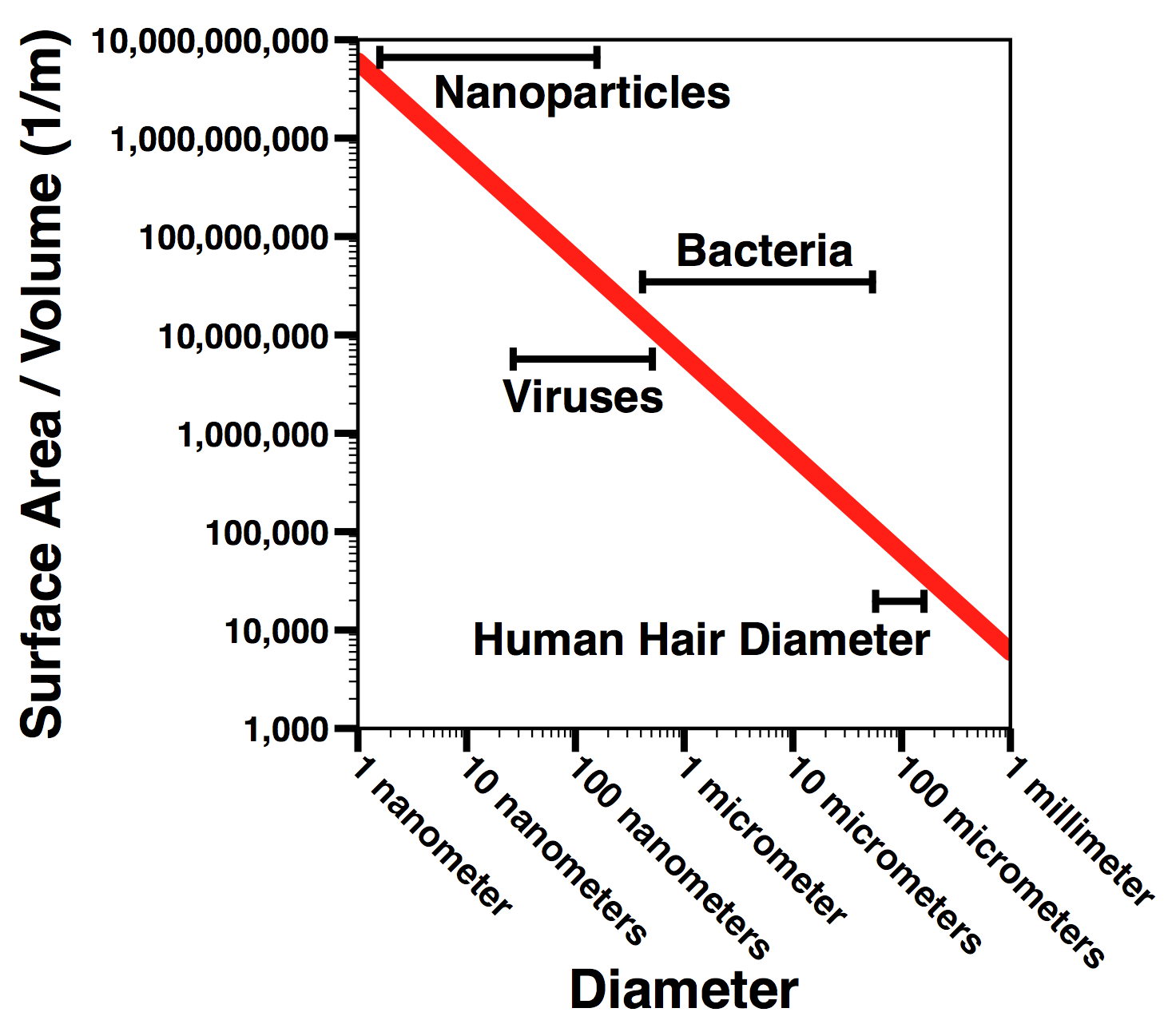
So how does this relate to nuclear power plants? Nuclear power plants are water-intense operations and rely on conductive heat transfer to convert nuclear energy to grid-ready electricity. The most common Western reactors are pressurized water reactors (PWRs) in which water is heated by pumping it through the reactor core, then pumping the hot water to a steam generator. This water flows through piping called the primary system and is kept in the liquid state by applying very high pressure through a device called a pressurizer. In the steam generator this primary system water transfers much of its heat to water in a secondary system. High-strength piping, which is a very effective heat conductor, keeps the water in the two systems from directly contacting each other. The secondary system’s water turns to steam when it absorbs the heat from the primary system. The steam is then directed via piping to drive a turbine, which turns an electric generator, thus completing the cycle of converting nuclear energy to readily usable electricity for the grid. After passing through the turbines, the steam is captured and condensed for recycling. This reclaimed water can then be sent back through the steam generator. However, a significant amount of the energy of this steam is lost to the atmosphere via a third system of cooling water that is used to condense the steam. Large amounts of water (in the form of water vapor) are released to the environment in this process. Think of the water vapor plume at the top of the iconic cooling towers seen in the cartoon TV show The Simpsons. (Not all nuclear power plants use these types of cooling towers, but all must emit heat to the environment through some means of cooling.)
A new class of nanomaterials called core-shell phase change nanoparticles may help in reducing the water loss. First, let’s parse the name of the nanoparticle. The core-shell nomenclature refers to the fact that the particle has a center made out of one material, and an outer skin made out of another material. The phase change component of the name refers to the fact that the particle center changes from a liquid to a solid under certain conditions. These particles may be mixed into the water used for transporting the thermal energy generated within the reactor. Once mixed into the reactor water, the particle cores melt as the water picks up thermal energy from the reactor. The melted material in the particle core is contained by a shell, which remains solid at reactor temperatures. Thus, as the water leaves the reactor it carries with it tiny particles containing bundles of liquid thermal energy wrapped in a solid core. The notion is that as these particles travel to the cooling tower, they solidify and dissipate their heat into the surrounding water, thus decreasing the amount of water needed to convert the thermal energy created by the reactor to steam for turning turbines. Additionally, since these particles do not vaporize, they are much more easily retained for recycling. The Electric Power Research Institute is currently working with scientists at Argonne National Laboratory to commercialize these particles and has suggested that this technology could decrease power plant water requirements by as much as 20 percent.5
Another nanoparticle-based approach for increasing reactor efficiency seeks to tackle a different problem. Pressurized water reactors place the water in direct contact with the fuel rods of the nuclear reactor. However, bubbles that form on the surfaces of the fuel rods can significantly decrease efficiency by insulating the rods from the water. When this happens, heat transfer efficiency suffers. One lab at the Massachusetts Institute of Technology (MIT) has implemented alumina nanoparticles that coat the fuel rods and prevent the buildup of bubbles on the heating elements. Alumina, a compound of aluminum and oxygen, is stable and has a high melting temperature. Testing these particles in the MIT reactor, the group found that the alumina nanoparticles coated the fuel rods. The result was an increase in the efficacy of the reactor. The engineers explain the findings by suggesting that the alumina nanoparticles allow for quick removal of the bubbles forming on fuel rod surfaces, thus minimizing the insulating layer of bubbles and maximizing heat transfer efficiency.6 To validate this, the researchers heated identical thin, steel wires a fraction of a millimeter in diameter. One wire was submerged in water, the other in a nanofluid containing alumina particles. The wires were heated to the point of boiling the surrounding fluid. After boiling the wires were examined using a powerful electron microscope. The experimenters observed that the wire heated in the nanofluid was indeed coated with nanoparticles, while the other wire maintained its original smooth surface.
Most importantly, there are also potential safety applications of having nanofluids capable of quickly transporting large quantities of thermal energy. One proposal calls for the use of nanofluids in standby coolant stored in Emergency Core Cooling Systems (ECCS). The ECCS are independent, standby systems designed to safely shut down a reactor in the case of an accident or malfunction. One ECCS component is a set of pumps and backup coolant to be sprayed directly onto reactor rods. Such systems are critical in preventing a loss of coolant accident (LOCA) from spiraling out of control. Because ECCS have backup reservoirs of coolant, technologies that make this backup coolant more effective at removing heat from the reactor could improve the safety of reactors. Because nanofluids can increase the heat transfer efficacy of water by 50 percent or more, some researchers have suggested that they may also be useful in emergency scenarios.7
Steam generators at both nuclear and coal power plants accounts for approximately 3 percent of overall freshwater consumption in the United States. Generally speaking, nuclear power plants consume about 400 gallons of water per megawatt-hour (MWh). Their coal and natural gas counterparts consume approximately 300 and 100 gallons per MWh, respectively.8 Thus, nuclear power plants stand to gain considerably by becoming more water-efficient.
However, there are many hurdles to tackle before nanoparticles can be safely and effectively used in operating power plants. Scaling up particle production to the large volumes of particles necessary for implementation in a power plant is expensive and labor intensive. New synthesis infrastructures may be necessary for large-scale production of these tiny particles. Additionally, broad adoption of this technology will not occur until significant cost savings are proven effective at a functioning plant. As a result, particles must be made available at a cost reasonable for adoption by power plant operators. A rough cost estimate can be made using commercially available alumina nanoparticles, as these particles have been tested extensively in the heat transfer literature. A typical nuclear power plant in the United States supplies enough electricity to power 740,000 homes. To do this, the plant requires between 13 and 23 gallons of water per home per day. Thus, water usages for the plant may range from 10 to 17 million gallons per day. Current vendors of aluminum oxide nanoparticles sell 1kg of nanopowder for around $200. With an expectation that economies of scale would bring that price down to $100/kg and that the particles could be easily recovered and recycled, loading a nuclear power plant with a 0.1% volume fraction of alumina nanoparticles would cost about $14.7 to $25 million per power plant. This is a substantial initial investment. Naturally, if nanoparticles were to cost $10/kg, then particle outfitting costs of $1.5 to $2.5 million per nuclear plant could be achieved. If 100 percent recovery of the particles could be achieved, then this initial cost would be recovered over time by the expected 2 to 4 percent increase in plant efficiency.
In addition to cost-benefit analysis, extensive testing must be performed to ensure long-term application of these particles does not threaten the operational safety of the plant. To accomplish this, smaller scale reactors (like those housed at research facilities and universities) may test these particles over the course of years to track the impacts of long-term use. Potential pitfalls include increased corrosion, system clogging, and nanoparticle leakage into wastewater. Corrosion engineers will be needed to validate the degree to which nanoparticles contribute to the overall aging of reactors in which they are used. Nanoparticle designers and hydrodynamicists will be needed to ensure that system clogging is manageable. Additionally, filtration experts and the Environmental Protection Agency will be needed to establish best practices for minimizing the amount of nanomaterial that exits the facility, as well as understanding and quantifying the environmental impacts of that emitted material. None of these potential roadblocks are trivial. However, while the challenges seem large, it is encouraging to see potential applications of nanotechnology in power plants.
A Looming Crisis of Confidence in Japan’s Nuclear Intentions
Nearly two years into Prime Minister Shinzo Abe’s second stint at governing Japan, his tenure has been characterized by three primary themes. The first two themes include his major legislative priorities: enabling Japan’s economic revival and bringing Japan closer to the status of a “normal” country that takes on a greater share of its own security needs. Both of these priorities are largely celebrated in the United States, which longs to see Japan become a more able and active partner in the region. A third theme has not been well received in Washington: the prime minister’s apparent efforts to whitewash Japan’s wartime past. Through personal expressions of admiration for convicted war-criminals, an official reinvestigation of past apologies for war-time atrocities, and appointments of hardline nationalists to prominent posts (such as the NHK board of governors), the prime minister’s actions have raised the spectre among wary neighbors of a Japanese return to militarism and begun raising eyebrows even among friends in Washington.
It is against this backdrop that Japan is now attempting to reinstate its nuclear energy program. Japan, which, not long ago, had planned to generate half of its electricity from nuclear power by 2030, has watched its nuclear reactors sit largely idle since the Fukushima disaster in 2011. Abe’s government views nuclear restarts as a critical pillar of his first legislative priority—Japan’s economic recovery. However, observers both outside and inside Japan note that, in addition to providing Japan the baseload electricity that its economy craves, the country’s sophisticated nuclear energy program effectively serves a dual purpose, providing Japan a latent nuclear weapons capability as well.
Citing Abe’s particular treatment of historical issues, some have begun to question whether reinstatement of Japan’s nuclear program is really more about the prime minister’s security goals than his economic agenda. China, for one, has hinted at allegations of a Japanese nuclear weapons program—after a recent incident in which Japan negotiated to repatriate an aging store of highly enriched uranium (HEU) to the United States, Chinese media propagated a narrative that twisted the event into evidence of Japan’s militaristic intentions.1 Koreans have begun expressing similar concerns.2
In fact, Japan’s current movement towards a more normal military posture is not entirely unrelated to the push to restart the country’s nuclear energy program—it was the Fukushima nuclear disaster that both idled Japan’s nuclear fleet and helped enable the return of the more hawkish LDP government. But the relationship likely ends there. As a legacy of World War II, Japanese society’s discomfort with the idea of a “normal” Japan has restricted Abe’s normalization efforts to steps that are only modest by any comparable measure.3 Events that have conspired to suggest the possibility of Japanese nuclear weapons are reflective of awkward timing and, perhaps, less than acute politics, but not likely of some new militant spirit in Japanese society. Unfortunately, as Japan pushes to restart its nuclear energy program in the months and years ahead, circumstances are aligning that will amplify—not mitigate—alarm over Japan’s nuclear intentions.
Japan’s Plutonium Economy
For a tangle of social and legal reasons, the restart of Japanese reactors is tied together with operation of Japan’s nuclear fuel reprocessing plant at Rokkasho Village in Aomori Prefecture. Under agreements with reactor host communities, utilities cannot operate reactors unless there is somewhere for nuclear fuel to go once it has been used. Because Japan lacks a geological repository and nearly all plant sites lack dry cask storage facilities,4 Rokkasho is currently the only viable destination for spent fuel from Japan’s reactors. Unless this situation changes, Japan is effectively unable to operate reactors without Rokkasho.
Rokkasho itself is, in turn, effectively dependent on Japan’s operating reactors. According to a sort of public-private arrangement that has been in place since before Fukushima, Japanese utilities send spent nuclear fuel to Rokkasho, where it is separated into waste and fissionable MOX (mixed uranium and plutonium oxides) powder. MOX is processed into fresh reactor fuel and sent back to Japan’s reactors. High-level waste is ultimately sent to a geological repository that is to be built in a different prefecture (one of Aomori Prefecture’s conditions for originally agreeing to host the reprocessing plant). Of Japan’s reactors, 16 to 18 of the 54 that were operating prior to the Fukushima accident would, after receiving local government consent, consume MOX in an effort to maximize use of Japan’s limited energy resources. That was the plan—prior to the Fukushima disaster, anyway.
As a legacy of the Fukushima disaster, Japan’s nuclear reactors currently sit idle. The six at Fukushima Daiichi will never operate again, nor will a number of others that are older, particularly vulnerable to earthquakes and tsunamis, or for other reasons not worth the trouble and expense of restarting. While impossible to predict for certain, a consensus seems to be emerging among experts and industry watchers that post-Fukushima, somewhere in the order of half of Japan’s original 54 reactors will return to service under Japan’s new regulatory regime. Currently, two reactors (Sendai 1 and 2 in southwestern Japan) have cleared safety reviews from Japan’s new regulator, the Japan Nuclear Regulatory Authority (JNRA), and now appear headed towards restart this winter. Eighteen more reactors await review from the JNRA. Of those 20 reactors, only five5 have received consent to use MOX, but that was prior to Fukushima. All 20 reactor restarts depend on the promise of a functioning Rokkasho. But if Rokkasho were to restart on a similar timeframe as the reactors, one thing is certain—there will be far fewer than the originally envisioned 16 to 18 reactors available to consume the MOX when the plant starts up.6
Reactor Restart X-Factors
As with Rokkasho, the question of when the JNRA will conclude its reviews of the next eighteen reactors remains quite murky. However, it stands to reason that ultimately most, if not all, of the reactors that have applied for restart will ultimately pass safety inspections. Japan’s electric power companies are unlikely to have invested the time and resources in plant upgrades and regulatory application had they less than a high degree of confidence that they would qualify under Japan’s new regime. Likewise, there is little question that Japan’s LDP government (assuming an LDP government at the time of restart) would stand in the way of restarts. But the JNRA and national government are only two of the three main factors in restarting Japan’s reactors—leaders of the towns and prefectures that host nuclear power plants have a de facto say in the matter as well.
The conventional wisdom is that local leaders have strong financial incentives to restart the nuclear power plants that they host: government and industry have historically lavished incentives on host communities and prefectures in order to overcome any inclination toward local resistance. In one sense, local governments have over time become dependent on plants and can ill afford to forego not only the government and utility incentives, but also the base of jobs and tax revenues they represent. On the other hand, communities need now only look to the example of the towns that have been rendered uninhabitable by the Fukushima disaster to see a terrifyingly clear picture of their tradeoff.
In some cases, apparently including the Sendai reactors, it is unlikely that local government would stand in the way of restarts. Earthquakes are less common in Kyushu,7 the geography on the west coast is less prone to large tsunamis, and local residents may take comfort in the fact that Sendai reactors are pressurized water reactors—not the boiling water rector type used at Fukushima Daiichi. But in other cases, local approvals may not be as certain. Take for example TEPCO’s Kashiwazaki-Kariwa plant in Nagano prefecture, where Governor Izumida has very publicly challenged TEPCO. He has insisted that, irrespective of the findings of the JNRA, with the Fukushima Daiichi reactor cores still too highly radioactive to investigate and verify the true nature of the accident, he will be unwilling to allow the Kashiwazaki-Kariwa reactors to restart.
In addition to the local government factor, an X-factor may be emerging—preemptive lawsuits against reactor restarts. Earlier this year, in Fukui prefecture where political leadership otherwise favors nuclear power, a citizens group brought a lawsuit alleging an inadequate basis for confidence in the restart of the Oi plant.8 More recently, a second lawsuit has been brought by the city of Hakodate (Hokkaido prefecture) against the yet-to-be completed Ohma plant in nearby Aomori prefecture.9 In the case of Oi, a local judge sided with the plaintiffs, but the decision has been appealed by Kansai Electric Power Company, and the case is all but certain to drag out until long past the serviceable lifetime of the Oi reactors. The Hakodate case is ongoing.
It is possible that Governor Izumida is an outlier and that the Fukui and Hakodate challenges will prove to be ineffective and isolated. However, it is equally possible that there are more Governor Izumidas and lawsuits yet to come. Furthermore, what is undeniable is that these cases have set a precedent and raised public pressure on local officials to seriously consider opposing restart of local reactors even if they do pass JNRA safety inspections. In any case, it is premature to presume that once the JNRA has rendered a safety verdict, reactor restart is imminent.
The MOX Question
Within the concurrent push to open Rokkasho and restart reactors, the availability of MOX-burning reactors seems to be assumed. But, notwithstanding all of the other hurdles facing nuclear reactor restarts in Japan, MOX fuel itself has been a subject of controversy and public discomfort since even before the Fukushima disaster. As utilities received approvals to burn MOX fuel and subsequently began receiving shipments of MOX from Europe (where it had been processed on behalf of Japan’s utilities), they were met with consistent and passionate public protests. These protests were typically confined to a cohort of smaller national-level interest groups that argue that using MOX elevates risk in transportation and regular reactor operation.10 On a national scale, prior to Fukushima the fuel cycle has been a relatively fringe issue—MOX had been a largely unfamiliar acronym to the public. Post-Fukushima, as utilities push for restarts amidst an atmosphere of heightened public scrutiny, there will be no free pass for MOX. For nuclear energy opponents, the prospect of MOX usage would provide one more narrative with which to hammer against proposed reactor restarts.
At the macro level, utilities share in the incentive to burn MOX fuel as they depend on Rokkasho, and Rokkasho is hard to rationalize in the absence of a functioning MOX program. However, in a much more tangible and immediate sense, utilities desperately need their reactors up and running again. Most of Japan’s utilities have posted consistent losses since their reactors were relegated to nonperforming assets on their balance sheets and they were forced to substitute expensive fossil fuels for relatively cheaper nuclear power. For Japan’s utilities, restarting nuclear reactors could be a life or death proposition. That being the case, can it be taken for granted that utilities will risk complicating their restart efforts by forging ahead with plans to burn MOX? Will the government create explicit incentive for utilities to do so? Given enhanced public scrutiny, it cannot be assumed that the pre-Fukushima local approvals for MOX usage will be honored anyway.
The Japanese government’s 2014 energy policy (despite reaffirming Japan’s commitment to its beleaguered ‘no surplus plutonium’ policy), gives blessing to proceeding with Rokkasho (recognizing that, among other things, if it didn’t, Aomori threatens to send the spent nuclear fuel right back to the plants of origin). But even assuming that the five MOX reactors under regulatory review do receive restart approval and recommence MOX burning, the original goal of 16 to 18 Japanese reactors burning MOX fuel seems far off.11 There has been some suggestion that Rokkasho could restart slowly, at a throughput commensurate with the ability to consume MOX. However, as Meiji University Professor Tadahiro Katsuta points out, reducing throughput of Rokkasho effectively raises the per-unit cost of MOX, necessitating a reexamination of the cost basis on which the MOX program was justified to Japanese ratepayers.12
Rokkasho Controversy
Even outside of the MOX capacity question, Rokkasho is not without controversy. Officially, Rokkasho is justified as an investment in energy security for Japan. However, from the standpoint of global nonproliferation concerns, Japan sets an uncomfortable precedent with Rokkasho. While otherwise a leading global champion for peace and nuclear disarmament, Japan is the only non-nuclear weapons country to possess a commercial nuclear fuel recycling program. Whereas global nonproliferation efforts prioritize limiting the spread of reprocessing capabilities, Rokkasho has enabled Iran, for one, to point to Japan in defending the legitimacy of its own fuel cycle activities. South Korea, seeking American consent for a Korean recycling program, also cites Japan’s example in negotiating a replacement for the U.S.-ROK nuclear cooperation agreement that expires in 2016.
Controversial or not, Japan’s leaders feel compelled to push forward with Rokkasho and through an agreement under section 123 of the Atomic Energy Act,13 they enjoy the support of the United States government. American consent to Rokkasho is only guaranteed through 2018, but the United States, which granted consent in 1988 largely out of deference to diplomatic concerns, for the same reason is highly unlikely to withdraw consent in 2018. Given the effective concurrence of the 2018 date with U.S.-ROK negotiations and the looming startup of Rokkasho in the face of low (or no) capacity to consume MOX, timing has become extremely awkward.
As Rokkasho proceeds towards restart, public reaction from Washington has been surprisingly muted. Perhaps this reflects appreciation for the energy conundrum in which Japan finds itself, or tacit consent that bringing Japan’s nuclear industry back onto solid footing after the Fukushima disaster was always going to be awkward—Japan has an inherent chicken or egg dilemma in restarting Rokkasho and its reactors. But the reality is that Japan’s situation puts Washington in a very tough spot. Washington is effectively complicit in what might appear to be Japanese disregard for its own commitments to global nonproliferation. This poses a risk to the global nonproliferation regime and American credibility on the subject.
Implications
Global nonproliferation principles undoubtedly remain a high priority for Japan. But it is likely that in the short term, the eyes of Japan’s leaders are focused more intently on bringing nuclear reactors back on line. Particularly in the context of Prime Minister Abe’s provocative views on history, the perception outside of Japan is certain to be one of alarm if Japan is seen to be separating plutonium without a credible pathway for its disposition. While the coincidence of the 2016/2018 Korea and Japan 123 agreements and Japan’s reentry into nuclear energy will shine a spotlight on the American role in Japan’s nuclear fuel cycle scheme, it is seen as highly unlikely that the United States will attempt to withdraw from or renegotiate the 123 agreement with Japan irrespective of Japan’s plutonium balance concerns. This will effectively make the United States appear complicit in Japan’s growing inventory of plutonium.
For the United States, this situation has consequences on three fronts. Firstly, Japan’s apparent failure to abide by its plutonium commitments undercuts American interests in limiting fuel cycle capabilities through treaty agreements. Nowhere is this more obvious than in the ongoing U.S.-ROK 123 agreement negotiations. Secondly, Japan is a leader, if not the symbolic face of the global nonproliferation regime. For Japan to be separating plutonium for no demonstrable purpose dramatically undercuts its own leadership on nonproliferation and aggravates the already controversial precedent it sets with its fuel cycle program, elevating the risk of proliferation in the region. Thirdly, at just the time when the United States is working to underscore its alliance with Japan as the bedrock of its security presence in East Asia, Japan’s growing plutonium surplus will only exacerbate concerns of Japan’s return to militarism, eroding its legitimacy and efficacy as a partner in regional security.
In the aftermath of the Fukushima disaster, the United States has appeared somewhat ambivalent in its response to Japan’s efforts to restart its nuclear energy system. However, the American stake in Japan’s road ahead is profound. While it is not the case in all foreign capitals, in Tokyo, opinions and preferences from Washington are meaningful. Washington, particularly the Department of State and Department of Energy, has an opportunity to protect American interests by formulating and articulating an unambiguous American position on Japan’s path forward on nuclear energy to Japan’s leadership.
The critical interest of the United States would be for Japan to demonstrate clear commitment to the no-surplus plutonium policy and to the global nonproliferation regime. As elements of a policy that might be necessary to make that happen, the United States should urge Japan’s leadership and utilities to:
- Articulate a plan for plutonium disposition that provides quantifiable and publicly demonstrable benchmarks for reducing Japan’s plutonium inventory.
- Call for official, temporary suspension of operations at Rokkasho until MOX burners or another credible disposition pathway for Japan’s separated fissile materials, is available.
- Advocate operating Rokkasho (if and when started), at an output rate that is no more than commensurate with plutonium disposition goals and available means for plutonium disposition.
- Encourage utilization of temporary dry-cask storage of spent nuclear fuel in order to enhance safety at reactor sites while expanding nuclear fuel cycle policy options. One of the rare positive stories to emerge from the Fukushima Daiichi disaster was the robustness of dry cask storage. Utilities and the government should capitalize on this success story and prioritize arrangements with local communities to allow for expeditious transfer of spent nuclear fuel from wet-storage to on-site dry casks.
There is no nuclear weapons program in Japan’s foreseeable future. However, there is a significant risk of an outward appearance that suggests otherwise to South Korea, China, North Korea, Iran, and the rest of the world. Whether or not appearance differs from reality, the real world consequences would likely be the same. While Japan has serious and immediate energy concerns, it also has a very deep and fundamental commitment to global nonproliferation. With support from friends in Washington, Japan must face its looming nuclear energy challenges head on with eyes fully open. The stakes are too high to allow current circumstances to dictate their own outcomes.
Next year is the 70th anniversary of the atomic bombings of Hiroshima and Nagasaki. The event would provide a fitting platform for Prime Minister Abe to recognize opportunities in Japan’s current crisis and make bold decisions on Japan’s nuclear energy program. The right decisions can help regain global confidence in Japan’s intentions, while reminding the world of Japan’s unwavering commitment to nuclear safety and nonproliferation. The anniversary would make an equally unfortunate occasion to demonstrate otherwise.
Ryan Shaffer is an Associate Director of Programs at the Maureen and Mike Mansfield Foundation in Washington, D.C., where he manages Japan and Northeast Asia policy programs including the Mansfield-FAS U.S.-Japan Nuclear Working Group. Prior to joining the Mansfield Foundation, Mr. Shaffer served as a research analyst for the Federation of Electric Power Companies of Japan.
Seismic Risk Management Solution for Nuclear Power Plants
Abstract
Nuclear power plants should safely operate during normal operations and maintain core-cooling capabilities during off-normal events, including external hazards (such as flooding and earthquakes). Management of external hazards to expectable levels of risk is critical to maintaining nuclear facility and nuclear power plant safety. Seismic risk is determined by convolving the seismic hazard with seismic fragilities (capacity of systems, structures, and components). Seismic isolation (SI) is one protective measure showing promise to minimize seismic risk.
Current SI designs (used in commercial industry) reduce horizontal earthquake loads and protect critical infrastructure from the potentially destructive effects of large earthquakes. The benefit of SI application in the nuclear industry is being recognized and SI systems have been proposed in American Society of Civil Engineer Standard 4, ASCE-4, to be released in the winter of 2014, for light water reactors facilities using commercially available technology. The intent of ASCE-4 is to provide criteria for seismic analysis of safety related nuclear structures such that the responses to design basis seismic events, computed in accordance with this standard, will have a small likelihood of being exceeded.
The U.S. nuclear industry has not implemented SI to date; a seismic isolation gap analysis meeting was convened on August 19, 2014, to determine progress on implementing SI in the U.S. nuclear industry. The meeting focused on the systems and components that could benefit from SI. This article highlights the gaps identified at this meeting.
Introduction
External hazards pose risks to nuclear power plants (NPPs) and nuclear facilities. Quantifying and managing this risk is important for safe operation of these facilities. Risk evaluations should follow a process similar to that shown in Figure 1; the process would start with risk-informed external hazard scenarios such as seismic, flood, fire, or tsunami, or a combination of these as initiating events. Verified and Validated (V&V) models would be used to simulate the external hazard initiators and model results would be used to determine which systems are at risk; decisions will be made on the protective measures needed to minimize risk. Seismic isolation is one protective measure showing promise to manage seismic risk.
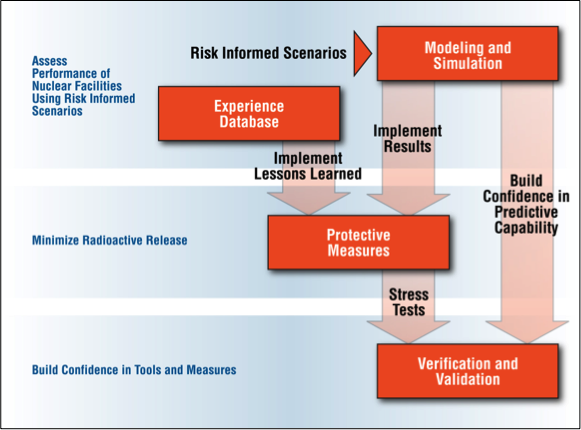
Future risk-informed process to minimize potential external hazard risk.
Seismic Isolation (SI) is gaining importance in the nuclear arena, especially after the Fukushima accident in 2011, which made the nuclear community even more aware that it must design and construct structures capable of withstanding large earthquakes (a previously alarming incident occurred in 2007; the Niigata Chuestu-Oki earthquake led to temporary shutdown of the Kashiwazaki-Kariwa NPP). Seismic base isolation has the potential to reduce horizontal earthquake loads for nuclear structures, systems, and components (SSCs). A substantial reduction in horizontal earthquake loading has the potential to increase the safety of nuclear SSCs by managing the risk associated with large seismic events. SI has been used for years in the non-nuclear commercial industry for buildings, bridges, liquid gas tanks, and offshore oil and gas platforms. In addition to SI systems for the current fleet of reactors, such as light water reactors (LWRs), there is a need for seismic isolation of nuclear facilities (both near-surface facilities and deeply embedded), related systems, and components such as diesel generators, reactor pressure vessels, steam generators, spent fuel pools, and critical response facilities. However, the seismic isolation solutions for LWRs (documented in ASCE-4) may not be appropriate for this purpose because the mass of many systems and components is relatively small and their geometry is very different.
The U.S. nuclear industry has not implemented SI to date. Therefore, a seismic isolation gap analysis meeting was convened on August 19, 2014, to determine progress on implementing SI in the U.S. nuclear industry, and what systems and components could benefit from SI. This working meeting included representatives from the Department of Energy (DOE), national laboratories, industry, Electric Power Research Institute (EPRI), and Nuclear Regulatory Commission (NRC) to discuss three SI topics: (1) general background on current SI progress in the United States, (2) limitations with implementing procedures outlined in ASCE-4 for SI solutions of entire nuclear power plants, and (3) potential SI solutions for systems and components, and gaps associated with developing standardized technologies, methods, and numerical tools for these solutions.
Seismic Isolation Need
Seismic hazard curves used in evaluating risk at nuclear facilities are continuously evolving as more information has been developed on seismic sources and seismic events. Moreover, additional research is performed to update attenuation relationships and characterize local site effects. Recent earthquake recordings at nuclear power plants sites have exceeded design basis values. These three recent earthquakes in the last 7 years are shown in Table 1. The seismic events were recorded at Kashiwazaki-Kariwa (TEPCO 1, 2007),1 Fukushima Daiichi and Fukushima Daini (TEPCO 2, March 2011),2 and North Anna (August 2011, detailed information provided in Virginia Electric and Power Company Memo).3

Peak recorded acceleration verses the design acceleration value.
The evolving nature of the seismic hazard curves and the requirement to reevaluate those curves every 10 years creates the possibility that a number of these seismic hazard curves will increase.4 Therefore, there is an opportunity for engineered solutions to manage this seismic uncertainty, and one solution is seismic isolation. SI has the potential to reduce horizontal earthquake loads for nuclear structures, systems, and components (SSCs). A substantial reduction in horizontal earthquake loading has the potential to increase the safety of nuclear SSCs by managing the risk associated with large seismic events. Installing horizontally flexible and vertically stiff seismic isolators between the superstructure and its foundation typically achieve isolation. Isolators serve two key functions: supporting gravity loads, and protecting the supported structure and its systems and components from the damaging effects of horizontal earthquake ground motion.
ASCE-4 provides standard procedures for providing robust seismic isolation systems for the entire NPP as shown in Figure 2. In addition to SI systems for LWRs (such as those shown in Figure 3), there is a need in the nuclear industry for seismic isolation of nuclear facility-related systems (both near surface facilities and deeply embedded facilities) and/or components (such as diesel generators, reactor pressure vessels, steam generators, spent fuel pools, and critical response facilities [such as FLEX support centers]).
FLEX is a strategy developed by the nuclear energy industry to implement the Nuclear Regulatory Commission (NRC)’s Fukushima task force recommendations5. FLEX provides backup electrical power and cooling capability if an extreme event impacts capability to maintain power and core cooling. One of the important aspects of FLEX is providing regional support centers that will provide emergency equipment to nuclear power plants during extreme events when necessary. FLEX support center locations are in Memphis, Tennessee and Phoenix, Arizona
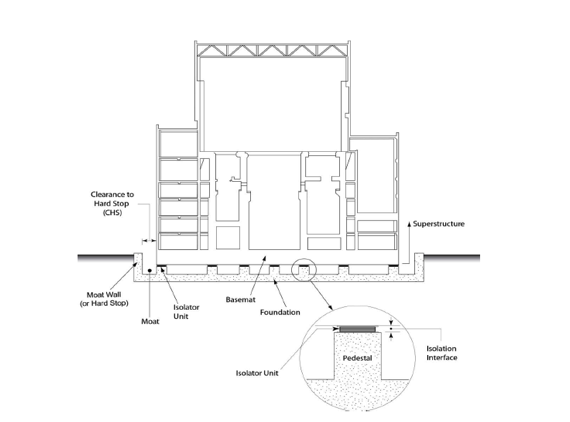
Proposed seismic isolation of LWR per ASCE-4.
The isolation solution shown in Figure 2 would not be cost effective for deeply embedded NPPs, since this would require over-excavation of the soil and installation of a moat system to accommodate the lateral displacement of the facility. Therefore, the likely benefit for deeply embedded structures would be systems and/or components internal to the NPP. For these systems and/or components, there may be a need to provide three-dimensional seismic isolation in addition to lateral SI. Due to the potential difference in the isolation environment (i.e., isolators may be located in the nuclear power plant instead of isolating the nuclear power plant) and the potential need to provide three-dimensional SI, it is necessary to provide required and improved numerical methods and consensus standards for SI of systems and/or components.
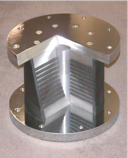
Seismic isolators: lead-rubber bearing (courtesy of DIS)
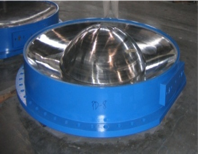
Seismic isolators: friction pendulum bearing (courtesy of EPS).
Seismic Isolation Gaps
The gaps identified in the working meeting fit into two areas: isolating the entire nuclear facility, and isolating systems and components. A systematic process was developed to determine the importance of each gap.
3.1 Entire Nuclear Facility
Hard Stop Cliff Edge Effects
The hard stop, as shown in Figure 2, is a concrete structure that limits the displacement of the installed seismic isolation system. The ASCE-4 committee has not addressed the potential cliff edge effects (and therefore unanalyzed damaged to the nuclear structures, systems, and/or components [SSCs]) during beyond design basis earthquake (BDBE). A cliff edge effect in a nuclear power plant is an instance of severely abnormal plant behavior caused by an abrupt transition from one plant status to another following a small deviation in a plant parameter, and thus a sudden large variation in plant conditions in response to a small variation in an input. So in the case of seismic isolation, the potential exists that during a large earthquake event the seismically isolated structure is appropriately decoupled from the ground motion; however, during the event a slightly larger displacement causes the structure to strike the hard stop. This impact could cause shock waves in the structure that damage critical components. This is an important parameter to consider in the SI application for a safe design.
Need: Medium
R&D would produce tools, methods, and technologies for addressing cliff edge effects. These would be implemented as guidance in industry consensus codes such as ASCE-4. Understanding of cliff edge effects (if any) and proposed solutions for design changes (if necessary) and demonstrating the reduction of in-structure nuclear facility response has potential to increase nuclear safety.
Hard Stop Requirements
Is the hard stop needed? Currently, ASCE-4 requires implementation of a hard stop to prevent displacement of the nuclear facility beyond what would cause tearing of the isolation system and catastrophic damage.
Need: High
Current state of knowledge is presented in ASCE-4, which requires implementation of the hard stop.
Seismic isolation methods and solutions for mitigating vertical seismic motion
SI of entire nuclear facility is standardized in a forthcoming version of ASCE-4. These are horizontal isolation systems. Vertical seismic response is an issue at some sites; there may be a need for vertical isolation. Currently, there is relatively little knowledge in the United States on designing robust vertical isolation. The need exists to understand the requirements, development methods, and solutions for vertical isolation.
Need: Medium
Vertical seismic response is an issue at some sites; a vertical isolation solution could manage the risk posed by large vertical ground motions.
Understanding margins in Seismic Analysis
Margins that may exist in seismic analysis are not clearly understood. Three recent earthquakes in the last 7 years have exceeded their design basis earthquake values (so damage to SSCs should have occurred), as shown in Table 1. However, seismic walk-downs at some of these plants indicate that very little damage occurred to safety class systems and components due to the seismic motion. Therefore, it is necessary to gather the applicable data to quantify the margins in current seismic analysis techniques (linear analysis) at nuclear power plants.
Need: High
Cost Benefit (Economic Viability) for SI of entire nuclear plant
To our knowledge, no one has performed a cost benefit analysis in the United States on an isolated versus non-isolated nuclear systems and components. A cost benefit analysis could provide valuable insight for venders interested in application of seismic isolation to manage seismic risk at their nuclear facilities.
Need: High
DOE and industry need to understand the cost benefit of implementing SI at NPPs.
Time Domain Methodology Requirements for Nonlinear Behavior
Industry needs a time domain approach that can handle nonlinear behavior, such as seismic isolation.
Need: Medium
Implementation of SI in Nuclear Facility Safety
The forthcoming version of ASCE 4 provides standardized language of implementation of SI for the entire nuclear facility. However, currently in the United States, SI related to nuclear facilities applications do not exist. After the Kashiwazaki-Kariwa earthquake in 2007, TEPCO installed seismically isolated emergency response buildings at all their NPP sites including Fukushima Daiichi (Hijikata 2012).6 These facilities provided valuable emergency response equipment to manage the nuclear reactor issues and allowed for continued onsite response.
Need: High
The consensus at the gap working meeting was that SI has the potential to manage seismic risk at NPP sites. One important comment from the meeting is there may be some reluctance in the nuclear community that first application of SI be the nuclear island. FLEX facilities, which support nuclear safety and other storage facilities, could be considered for SI application to demonstrate implementation of the technology in the United States nuclear safety arena.
3.2 Systems and Components
SI Solutions for Nuclear Systems and Components
Seismic isolation solutions for LWRs (documented in ASCE-4) may not be appropriate for isolation of systems and/or components because the mass of many systems and/or components is relatively small and their geometry is very different. Can SI solutions presented in ASCE-4 be scaled for implementation with systems and components, or is it necessary to develop new SI solutions?
Need: High
Deeply embedded NPP venders are likely to seek SI solutions (if needed) for systems and components. Currently, ASCE 4 does not address SI of systems and components; therefore, development of SI solutions for systems and components is necessary. Implementation of these solutions on consensus codes is necessary.
SI for Individual Components, Leading to Differential Displacement Issues
Differential displacement between the isolated and unisolated interface is a known issue that should be addressed in the design. It is also important to quantify the risk associated with this differential displacement.
Need: Medium
Model Validation and Uncertainty Quantification
SI numerical models must be verified and validated to provide confidence in predicted structural response. Nonlinear structural dynamics models are needed to accurately predict the behavior of seismic isolation systems. Uncertainty quantification is essential to accessing the range of validity for the model (based on assumptions) and experiments (based on operating conditions).
Need: Medium
Rocking Effect on Seismic Isolation of a Component (essential to understand for Integral SMR designs)
SI of certain NPP systems and components may create rocking loads that current isolation technologies (standardized in ASCE-4), were not designed to handle. SI solutions for deeply embedded structures will likely only be used for systems and components. Therefore, it is necessary to develop SI tools, methods, and solutions similar to what is currently in ASCE 4 for SI of the entire NPP.
Need: Medium
Depending on SI designs for systems and components, this may be important.
Results and Conclusions
Understanding the impact external hazards, such as flooding and earthquakes, can have on nuclear facilities and nuclear power plants is critical to deciding how to manage these hazards to expectable levels of risk. Seismic isolation offers the potential to manage nuclear power plant risk associated with large seismic events. An incremental step in implementing SI in the nuclear arena, prior to actually isolating some portion of a nuclear plant, could be an application for consolidated used fuel facilities, or the two FLEX locations in Memphis, Tennessee and Phoenix, Arizona, which are the two U.S. storage locations for spare diesel generators, pumps, etc., selected by industry in response to Fukushima.
The gaps and research and development needed to close these gaps are identified in Coleman and Sabharwall, 2014 (INL-14-33234).7This should be used to guide the future requirements for the research communities and industry. The gaps that are identified as most important are:
- SI solutions for nuclear systems and components
- Quantification of cost benefit (economic viability) for SI
- Understanding margins in seismic analysis
- Implementation of SI in nuclear facility
Implementation of seismic isolation at nuclear power plants and critical facilities has the potential to minimize risk at nuclear facilities associated with large seismic motions, and reduce the cost of construction. Therefore, it is necessary to perform research and development activities that focus on collaborative efforts between, industry, national laboratories, and universities to close the gaps that are identified.
Justin Coleman is a Civil/Structural Engineer at Idaho National Laboratory (INL). He performs seismic and structural dynamics research at INL and has a master’s degree in engineering structures and mechanics. Mr. Coleman’s background and expertise is seismic analysis of nuclear facilities and impact analysis of spent fuel casks. Much of the impact analysis work is performed using explicit finite element analysis codes. He has performed linear and nonlinear seismic soil-structure interaction (SSI) analysis for safety-related nuclear structures and is currently working to develop advanced nonlinear seismic soil-structure interaction analysis methodologies. His research interests include nonlinear SSI analysis, advanced seismic PRA, seismic protective systems, spend fuel transportation and storage, and beyond design basis threats to nuclear structures. He serves on the ASCE 4 and 43 where he is the lead author of Chapter 3, “Modeling of Structures,” and led the effort to write Appendix B, “Nonlinear Time Domain Soil-Structure Interaction Analysis,” in the forthcoming edition of ASCE 4. Mr. Coleman has authored numerous reports on impact analysis of spent fuel casks and nuclear fuel, and seismic analysis.
Dr. Piyush Sabharwall is a staff research scientist in Nuclear System Design and Analysis Division at Idaho National Laboratory (INL). He has a premier role in the development of very high temperature nuclear reactor technologies that are integral to the Department of Energy (DOE) strategic plans for the sustained advances in nuclear energy. Dr. Sabharwall has expertise in heat transfer, fluid mechanics, thermal design, thermodynamics, and nuclear safety analyses. Over the last few years, he has been researching on high temperature heat exchanger design & optimization, system integrations & power conversion systems and safety and reliability for Advanced Reactor Concepts. He obtained his Masters in Nuclear Engineering with a minor in Mechanical Engineering from Oregon State University and further pursued his Doctorate in Nuclear Engineering from University of Idaho. He has authored over 75 publications including journal articles, conference proceedings, technical abstracts, magazines and technical reports and has acted as a reviewer for journal and conference proceedings. He has been a significant catalyst for international and national research partnerships in the field of thermal hydraulics (heat and mass transfer, fluid mechanics, two phase flow, computational multiphase flow technology) for both nuclear and mechanical industries. In 2011 he received the New Faces of Engineering ASME National Award on the basis of contributions stemming from experiments and research and development effort in the area of thermal hydraulics and in 2013 he was awarded the ANS Young Member Excellence National Award.