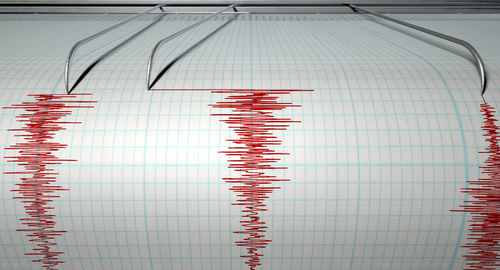
Seismic Risk Management Solution for Nuclear Power Plants
Abstract
Nuclear power plants should safely operate during normal operations and maintain core-cooling capabilities during off-normal events, including external hazards (such as flooding and earthquakes). Management of external hazards to expectable levels of risk is critical to maintaining nuclear facility and nuclear power plant safety. Seismic risk is determined by convolving the seismic hazard with seismic fragilities (capacity of systems, structures, and components). Seismic isolation (SI) is one protective measure showing promise to minimize seismic risk.
Current SI designs (used in commercial industry) reduce horizontal earthquake loads and protect critical infrastructure from the potentially destructive effects of large earthquakes. The benefit of SI application in the nuclear industry is being recognized and SI systems have been proposed in American Society of Civil Engineer Standard 4, ASCE-4, to be released in the winter of 2014, for light water reactors facilities using commercially available technology. The intent of ASCE-4 is to provide criteria for seismic analysis of safety related nuclear structures such that the responses to design basis seismic events, computed in accordance with this standard, will have a small likelihood of being exceeded.
The U.S. nuclear industry has not implemented SI to date; a seismic isolation gap analysis meeting was convened on August 19, 2014, to determine progress on implementing SI in the U.S. nuclear industry. The meeting focused on the systems and components that could benefit from SI. This article highlights the gaps identified at this meeting.
Introduction
External hazards pose risks to nuclear power plants (NPPs) and nuclear facilities. Quantifying and managing this risk is important for safe operation of these facilities. Risk evaluations should follow a process similar to that shown in Figure 1; the process would start with risk-informed external hazard scenarios such as seismic, flood, fire, or tsunami, or a combination of these as initiating events. Verified and Validated (V&V) models would be used to simulate the external hazard initiators and model results would be used to determine which systems are at risk; decisions will be made on the protective measures needed to minimize risk. Seismic isolation is one protective measure showing promise to manage seismic risk.
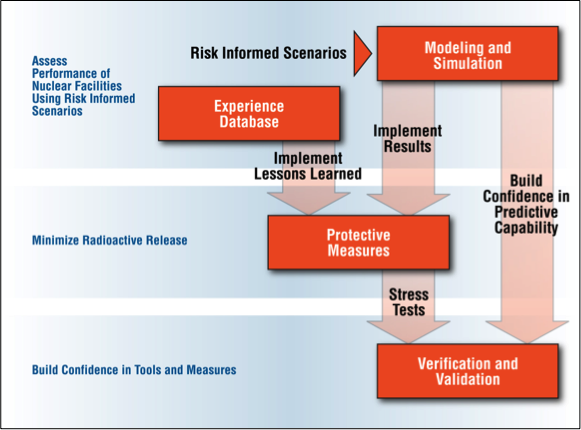
Future risk-informed process to minimize potential external hazard risk.
Seismic Isolation (SI) is gaining importance in the nuclear arena, especially after the Fukushima accident in 2011, which made the nuclear community even more aware that it must design and construct structures capable of withstanding large earthquakes (a previously alarming incident occurred in 2007; the Niigata Chuestu-Oki earthquake led to temporary shutdown of the Kashiwazaki-Kariwa NPP). Seismic base isolation has the potential to reduce horizontal earthquake loads for nuclear structures, systems, and components (SSCs). A substantial reduction in horizontal earthquake loading has the potential to increase the safety of nuclear SSCs by managing the risk associated with large seismic events. SI has been used for years in the non-nuclear commercial industry for buildings, bridges, liquid gas tanks, and offshore oil and gas platforms. In addition to SI systems for the current fleet of reactors, such as light water reactors (LWRs), there is a need for seismic isolation of nuclear facilities (both near-surface facilities and deeply embedded), related systems, and components such as diesel generators, reactor pressure vessels, steam generators, spent fuel pools, and critical response facilities. However, the seismic isolation solutions for LWRs (documented in ASCE-4) may not be appropriate for this purpose because the mass of many systems and components is relatively small and their geometry is very different.
The U.S. nuclear industry has not implemented SI to date. Therefore, a seismic isolation gap analysis meeting was convened on August 19, 2014, to determine progress on implementing SI in the U.S. nuclear industry, and what systems and components could benefit from SI. This working meeting included representatives from the Department of Energy (DOE), national laboratories, industry, Electric Power Research Institute (EPRI), and Nuclear Regulatory Commission (NRC) to discuss three SI topics: (1) general background on current SI progress in the United States, (2) limitations with implementing procedures outlined in ASCE-4 for SI solutions of entire nuclear power plants, and (3) potential SI solutions for systems and components, and gaps associated with developing standardized technologies, methods, and numerical tools for these solutions.
Seismic Isolation Need
Seismic hazard curves used in evaluating risk at nuclear facilities are continuously evolving as more information has been developed on seismic sources and seismic events. Moreover, additional research is performed to update attenuation relationships and characterize local site effects. Recent earthquake recordings at nuclear power plants sites have exceeded design basis values. These three recent earthquakes in the last 7 years are shown in Table 1. The seismic events were recorded at Kashiwazaki-Kariwa (TEPCO 1, 2007),1 Fukushima Daiichi and Fukushima Daini (TEPCO 2, March 2011),2 and North Anna (August 2011, detailed information provided in Virginia Electric and Power Company Memo).3

Peak recorded acceleration verses the design acceleration value.
The evolving nature of the seismic hazard curves and the requirement to reevaluate those curves every 10 years creates the possibility that a number of these seismic hazard curves will increase.4 Therefore, there is an opportunity for engineered solutions to manage this seismic uncertainty, and one solution is seismic isolation. SI has the potential to reduce horizontal earthquake loads for nuclear structures, systems, and components (SSCs). A substantial reduction in horizontal earthquake loading has the potential to increase the safety of nuclear SSCs by managing the risk associated with large seismic events. Installing horizontally flexible and vertically stiff seismic isolators between the superstructure and its foundation typically achieve isolation. Isolators serve two key functions: supporting gravity loads, and protecting the supported structure and its systems and components from the damaging effects of horizontal earthquake ground motion.
ASCE-4 provides standard procedures for providing robust seismic isolation systems for the entire NPP as shown in Figure 2. In addition to SI systems for LWRs (such as those shown in Figure 3), there is a need in the nuclear industry for seismic isolation of nuclear facility-related systems (both near surface facilities and deeply embedded facilities) and/or components (such as diesel generators, reactor pressure vessels, steam generators, spent fuel pools, and critical response facilities [such as FLEX support centers]).
FLEX is a strategy developed by the nuclear energy industry to implement the Nuclear Regulatory Commission (NRC)’s Fukushima task force recommendations5. FLEX provides backup electrical power and cooling capability if an extreme event impacts capability to maintain power and core cooling. One of the important aspects of FLEX is providing regional support centers that will provide emergency equipment to nuclear power plants during extreme events when necessary. FLEX support center locations are in Memphis, Tennessee and Phoenix, Arizona
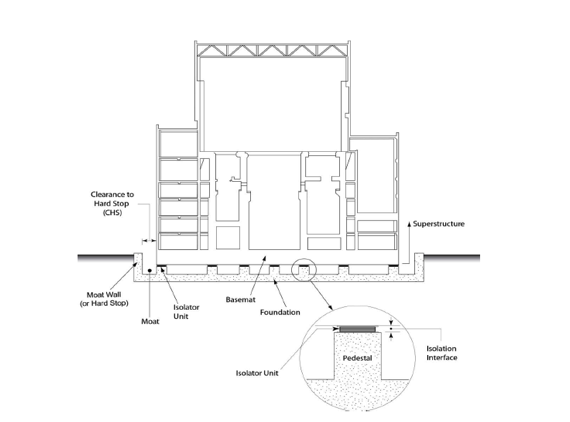
Proposed seismic isolation of LWR per ASCE-4.
The isolation solution shown in Figure 2 would not be cost effective for deeply embedded NPPs, since this would require over-excavation of the soil and installation of a moat system to accommodate the lateral displacement of the facility. Therefore, the likely benefit for deeply embedded structures would be systems and/or components internal to the NPP. For these systems and/or components, there may be a need to provide three-dimensional seismic isolation in addition to lateral SI. Due to the potential difference in the isolation environment (i.e., isolators may be located in the nuclear power plant instead of isolating the nuclear power plant) and the potential need to provide three-dimensional SI, it is necessary to provide required and improved numerical methods and consensus standards for SI of systems and/or components.
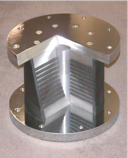
Seismic isolators: lead-rubber bearing (courtesy of DIS)
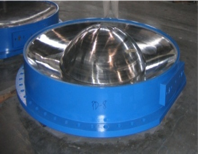
Seismic isolators: friction pendulum bearing (courtesy of EPS).
Seismic Isolation Gaps
The gaps identified in the working meeting fit into two areas: isolating the entire nuclear facility, and isolating systems and components. A systematic process was developed to determine the importance of each gap.
3.1 Entire Nuclear Facility
Hard Stop Cliff Edge Effects
The hard stop, as shown in Figure 2, is a concrete structure that limits the displacement of the installed seismic isolation system. The ASCE-4 committee has not addressed the potential cliff edge effects (and therefore unanalyzed damaged to the nuclear structures, systems, and/or components [SSCs]) during beyond design basis earthquake (BDBE). A cliff edge effect in a nuclear power plant is an instance of severely abnormal plant behavior caused by an abrupt transition from one plant status to another following a small deviation in a plant parameter, and thus a sudden large variation in plant conditions in response to a small variation in an input. So in the case of seismic isolation, the potential exists that during a large earthquake event the seismically isolated structure is appropriately decoupled from the ground motion; however, during the event a slightly larger displacement causes the structure to strike the hard stop. This impact could cause shock waves in the structure that damage critical components. This is an important parameter to consider in the SI application for a safe design.
Need: Medium
R&D would produce tools, methods, and technologies for addressing cliff edge effects. These would be implemented as guidance in industry consensus codes such as ASCE-4. Understanding of cliff edge effects (if any) and proposed solutions for design changes (if necessary) and demonstrating the reduction of in-structure nuclear facility response has potential to increase nuclear safety.
Hard Stop Requirements
Is the hard stop needed? Currently, ASCE-4 requires implementation of a hard stop to prevent displacement of the nuclear facility beyond what would cause tearing of the isolation system and catastrophic damage.
Need: High
Current state of knowledge is presented in ASCE-4, which requires implementation of the hard stop.
Seismic isolation methods and solutions for mitigating vertical seismic motion
SI of entire nuclear facility is standardized in a forthcoming version of ASCE-4. These are horizontal isolation systems. Vertical seismic response is an issue at some sites; there may be a need for vertical isolation. Currently, there is relatively little knowledge in the United States on designing robust vertical isolation. The need exists to understand the requirements, development methods, and solutions for vertical isolation.
Need: Medium
Vertical seismic response is an issue at some sites; a vertical isolation solution could manage the risk posed by large vertical ground motions.
Understanding margins in Seismic Analysis
Margins that may exist in seismic analysis are not clearly understood. Three recent earthquakes in the last 7 years have exceeded their design basis earthquake values (so damage to SSCs should have occurred), as shown in Table 1. However, seismic walk-downs at some of these plants indicate that very little damage occurred to safety class systems and components due to the seismic motion. Therefore, it is necessary to gather the applicable data to quantify the margins in current seismic analysis techniques (linear analysis) at nuclear power plants.
Need: High
Cost Benefit (Economic Viability) for SI of entire nuclear plant
To our knowledge, no one has performed a cost benefit analysis in the United States on an isolated versus non-isolated nuclear systems and components. A cost benefit analysis could provide valuable insight for venders interested in application of seismic isolation to manage seismic risk at their nuclear facilities.
Need: High
DOE and industry need to understand the cost benefit of implementing SI at NPPs.
Time Domain Methodology Requirements for Nonlinear Behavior
Industry needs a time domain approach that can handle nonlinear behavior, such as seismic isolation.
Need: Medium
Implementation of SI in Nuclear Facility Safety
The forthcoming version of ASCE 4 provides standardized language of implementation of SI for the entire nuclear facility. However, currently in the United States, SI related to nuclear facilities applications do not exist. After the Kashiwazaki-Kariwa earthquake in 2007, TEPCO installed seismically isolated emergency response buildings at all their NPP sites including Fukushima Daiichi (Hijikata 2012).6 These facilities provided valuable emergency response equipment to manage the nuclear reactor issues and allowed for continued onsite response.
Need: High
The consensus at the gap working meeting was that SI has the potential to manage seismic risk at NPP sites. One important comment from the meeting is there may be some reluctance in the nuclear community that first application of SI be the nuclear island. FLEX facilities, which support nuclear safety and other storage facilities, could be considered for SI application to demonstrate implementation of the technology in the United States nuclear safety arena.
3.2 Systems and Components
SI Solutions for Nuclear Systems and Components
Seismic isolation solutions for LWRs (documented in ASCE-4) may not be appropriate for isolation of systems and/or components because the mass of many systems and/or components is relatively small and their geometry is very different. Can SI solutions presented in ASCE-4 be scaled for implementation with systems and components, or is it necessary to develop new SI solutions?
Need: High
Deeply embedded NPP venders are likely to seek SI solutions (if needed) for systems and components. Currently, ASCE 4 does not address SI of systems and components; therefore, development of SI solutions for systems and components is necessary. Implementation of these solutions on consensus codes is necessary.
SI for Individual Components, Leading to Differential Displacement Issues
Differential displacement between the isolated and unisolated interface is a known issue that should be addressed in the design. It is also important to quantify the risk associated with this differential displacement.
Need: Medium
Model Validation and Uncertainty Quantification
SI numerical models must be verified and validated to provide confidence in predicted structural response. Nonlinear structural dynamics models are needed to accurately predict the behavior of seismic isolation systems. Uncertainty quantification is essential to accessing the range of validity for the model (based on assumptions) and experiments (based on operating conditions).
Need: Medium
Rocking Effect on Seismic Isolation of a Component (essential to understand for Integral SMR designs)
SI of certain NPP systems and components may create rocking loads that current isolation technologies (standardized in ASCE-4), were not designed to handle. SI solutions for deeply embedded structures will likely only be used for systems and components. Therefore, it is necessary to develop SI tools, methods, and solutions similar to what is currently in ASCE 4 for SI of the entire NPP.
Need: Medium
Depending on SI designs for systems and components, this may be important.
Results and Conclusions
Understanding the impact external hazards, such as flooding and earthquakes, can have on nuclear facilities and nuclear power plants is critical to deciding how to manage these hazards to expectable levels of risk. Seismic isolation offers the potential to manage nuclear power plant risk associated with large seismic events. An incremental step in implementing SI in the nuclear arena, prior to actually isolating some portion of a nuclear plant, could be an application for consolidated used fuel facilities, or the two FLEX locations in Memphis, Tennessee and Phoenix, Arizona, which are the two U.S. storage locations for spare diesel generators, pumps, etc., selected by industry in response to Fukushima.
The gaps and research and development needed to close these gaps are identified in Coleman and Sabharwall, 2014 (INL-14-33234).7This should be used to guide the future requirements for the research communities and industry. The gaps that are identified as most important are:
- SI solutions for nuclear systems and components
- Quantification of cost benefit (economic viability) for SI
- Understanding margins in seismic analysis
- Implementation of SI in nuclear facility
Implementation of seismic isolation at nuclear power plants and critical facilities has the potential to minimize risk at nuclear facilities associated with large seismic motions, and reduce the cost of construction. Therefore, it is necessary to perform research and development activities that focus on collaborative efforts between, industry, national laboratories, and universities to close the gaps that are identified.
Justin Coleman is a Civil/Structural Engineer at Idaho National Laboratory (INL). He performs seismic and structural dynamics research at INL and has a master’s degree in engineering structures and mechanics. Mr. Coleman’s background and expertise is seismic analysis of nuclear facilities and impact analysis of spent fuel casks. Much of the impact analysis work is performed using explicit finite element analysis codes. He has performed linear and nonlinear seismic soil-structure interaction (SSI) analysis for safety-related nuclear structures and is currently working to develop advanced nonlinear seismic soil-structure interaction analysis methodologies. His research interests include nonlinear SSI analysis, advanced seismic PRA, seismic protective systems, spend fuel transportation and storage, and beyond design basis threats to nuclear structures. He serves on the ASCE 4 and 43 where he is the lead author of Chapter 3, “Modeling of Structures,” and led the effort to write Appendix B, “Nonlinear Time Domain Soil-Structure Interaction Analysis,” in the forthcoming edition of ASCE 4. Mr. Coleman has authored numerous reports on impact analysis of spent fuel casks and nuclear fuel, and seismic analysis.
Dr. Piyush Sabharwall is a staff research scientist in Nuclear System Design and Analysis Division at Idaho National Laboratory (INL). He has a premier role in the development of very high temperature nuclear reactor technologies that are integral to the Department of Energy (DOE) strategic plans for the sustained advances in nuclear energy. Dr. Sabharwall has expertise in heat transfer, fluid mechanics, thermal design, thermodynamics, and nuclear safety analyses. Over the last few years, he has been researching on high temperature heat exchanger design & optimization, system integrations & power conversion systems and safety and reliability for Advanced Reactor Concepts. He obtained his Masters in Nuclear Engineering with a minor in Mechanical Engineering from Oregon State University and further pursued his Doctorate in Nuclear Engineering from University of Idaho. He has authored over 75 publications including journal articles, conference proceedings, technical abstracts, magazines and technical reports and has acted as a reviewer for journal and conference proceedings. He has been a significant catalyst for international and national research partnerships in the field of thermal hydraulics (heat and mass transfer, fluid mechanics, two phase flow, computational multiphase flow technology) for both nuclear and mechanical industries. In 2011 he received the New Faces of Engineering ASME National Award on the basis of contributions stemming from experiments and research and development effort in the area of thermal hydraulics and in 2013 he was awarded the ANS Young Member Excellence National Award.
The current planned capacity for lithium processing in the U.S. is on track to meet demand from domestic battery factories. However, current planned capacity for nickel, cobalt, and graphite still fall well short of future demand.
Dr. Pierre-Clément Simon and Dr. Casey Icenhour come from different backgrounds, but share similar passions: for driving forward progress in fusion energy and mentoring early career scientists.
As emerging clean energy technologies move along the innovation pipeline from first concept to commercialization, they encounter hurdles that can prove to be a death knell for young startups.
As ‘Friends of FESI’ we want to see this new foundation set up from day one to successfully fulfill the promise of its large impact.