Reflections on the 70th Anniversary of the Manhattan Project: Questions and Answers
I began my professional life by obtaining degrees in physics and entering a conventional academic career in teaching and astronomical research, but I had always been curious about the physics of the Manhattan Project and its role in ending World War II. With grants, publications and tenure established, I began to indulge this interest as a legitimate part of my work and about 20 years ago, to explore it in depth.
As anybody that comes to this topic in more than a casual way will attest, it can grow into an obsession. I have now published two books on the Project, well over two dozen articles and book reviews in technical, historical, and semi-popular journals, and have made a number of presentations at professional conferences. Over this time I must have looked at thousands of archived documents and held hundreds of real and electronic conversations with other scientists, historians, and writers whose interest in this pivotal event parallels my own. While my knowledge of the Project is certainly not and never will be complete, I have learned much about it over the last 20 years.
To my surprise (and pleasure) I am frequently asked questions about the Project by students, family members, guests at dinner parties, colleagues at American Physical Society meetings, and even casual acquaintances at my favorite coffee shop. Typical queries are:
“Why did we drop the bombs? Were they necessary to end the war?”
“Did President Truman and his advisors really understand the power of the bombs and the destruction they could cause?”
“Have nuclear weapons helped deter subsequent large-scale wars, and do we still need a deterrent?”
“What about the ethical aspects?”
“In studying the Manhattan Project, what most surprised you? Do you think it or something similar could be done now?”
At first I was awkward in trying to answer these questions but with passing years, increased knowledge, and much reflection I now feel more comfortable addressing them. With accumulating experience in a scientific career, you often learn that the questions you and others initially thought to be important may not be the ones that the facts address and that there may be much more interesting issues behind the obvious ones. In this spirit, I offer in this essay some very personal reflections on the Project and the legacies of Hiroshima and Nagasaki, framed as responses to questions like those above. In some cases a “yes” or “no” along with an explanation will do, but for many issues the nuances involved obviate a simple response.
I begin with the issue of the “decision” to use the bomb and the state of President Truman’s knowledge. In the spring of 1945, Secretary of War Henry Stimson assembled a committee to consider and advise upon immediate and long-term aspects of atomic energy. This “Interim Committee” comprised eight civilians, including three scientists intimately familiar with the Manhattan Project: Vannevar Bush, James Conant, and Karl Compton. In a meeting on May 31 which was attended by Army Chief of Staff General George C. Marshall, Stimson opened with a statement as to how he viewed the significance of the Project1:
The Secretary expressed the view, a view shared by General Marshall, that this project should not be considered simply in terms of military weapons, but as a new relationship of man to the universe. This discovery might be compared to the discoveries of the Copernican theory and of the laws of gravity, but far more important than these in its effect on the lives of men. While the advances in the field to date had been fostered by the needs of war, it was important to realize that the implications of the project went far beyond the needs of the present war. It must be controlled if possible to make it an assurance of future peace rather than a menace to civilization.
For his part, President Truman had been thoroughly briefed on the project by Stimson and General Leslie Groves, director of the Project, soon after he became President in late April. In late July, Truman recorded his reaction to the Trinity test in his diary2:
We have discovered the most terrible bomb in the history of the world. … Anyway we think we have found the way to cause a disintegration of the atom. An experiment in the New Mexico desert was startling – to put it mildly. Thirteen pounds of the explosive caused the complete disintegration of a steel tower 60 feet high, created a crater 6 feet deep and 1,200 feet in diameter, knocked over a steel tower 1/2 mile away and knocked men down 10,000 yards away. The explosion was visible for more than 200 miles and audible for 40 miles and more. … The target will be a purely military one and we will issue a warning statement asking the Japs to surrender and save lives. I’m sure they will not do that, but we will have given them the chance. It is certainly a good thing for the world that Hitler’s crowd or Stalin’s did not discover this atomic bomb. It seems to be the most terrible thing ever discovered, but it can be made the most useful…
I have no doubt that Stimson, Marshall and Truman were well aware of the revolutionary nature of the bomb and the possibility (indeed, likelihood) that a postwar nuclear arms race would ensue. Any notion that Truman was a disengaged observer carried along by the momentum of events is hard to believe in view of the above comments. These men were making decisions of grave responsibility and were fully briefed as to both the immediate situation of the war and possible long-term geopolitical consequences: the “mature consideration” that Franklin Roosevelt and Winston Churchill agreed in 1943 would have to be carried out before use of the bombs was authorized. Perhaps Truman did not so much make a positive decision to use the bombs so much as he opted not to halt operations that were already moving along when he became President, but I have no doubt that he realized that atomic bombs would be a profoundly new type of weapon. Further, let us not forget that it was Truman who personally intervened after Nagasaki to order a halt to further atomic bombings when the Japanese began to signal a willingness to consider surrender negotiations.
As much as I am convinced that Truman took his duties with the greatest sense of responsibility, I cannot answer “yes” or “no” as to the necessity of the bombings: the question is always loaded with so many unstated perspectives. If the Japanese could not be convinced to surrender, then Truman, Stimson, and Marshall faced the prospect of committing hundreds of thousands of men to a horrific invasion followed by a likely even more horrific slog through the Japanese home islands. After 70 years it is easy to forget the context of the war in the summer of 1945. Historians know that the Japanese were seeking a path to honorable surrender and might have given up within a few weeks, but the very bloody fact on the ground was that they had not yet surrendered; thousands of Allied and Japanese servicemen were dying each week in the Pacific. Military historian Dennis Giangreco has studied Army and War Department manpower projections for the two-part invasion of Japan scheduled for late 1945 and the spring of 19463. Planning was based on having to sustain an average of 100,000 casualties per month from November 1945 through the fall of 1946. The invasion of Kyushu was scheduled to begin on November 1, 1945. Had this occurred, the number of casualties might well have exceeded the number of deaths at Hiroshima and Nagasaki, let alone those which would have occurred in the meantime. From the perspective of preventing casualties, perhaps it was unfortunate that the bombs were not ready at the time of the battle for Iwo Jima, one of the bloodiest protracted battles from February 19 to March 26, 1945, during which more than 25,000 were killed on both sides.
Even if they believe that the Soviet Union’s declaration of war on the night of August 8, 1945, against Japan was the most significant factor in the Japanese decision to surrender, most historians allow that the bombs had at least some effect on that decision. The Soviet invasion came between the two atomic bombings on August 6 (Hiroshima) and August 9 (Nagasaki). These two bombings would convince the Japanese that Hiroshima was not a one-shot deal: America could manufacture atomic bombs in quantity. The impact of the bombings was alluded to by Emperor Hirohito in his message to his people on August 15, 1945, in which he stated that “ … the enemy has begun to employ a new and most cruel bomb,” which was one of the motivations for his government’s decision to accept the terms of the Potsdam Declaration. But there are certainly political aspects that muddy this story, namely justifying the immense resources poured into the Project and sending a message to the Soviets that at least for a while America was the ascendant postwar power in the world. I give a qualified “yes” to the question of necessity.
The necessity debate often overlooks a corollary issue which I have come to think of as “nuclear inoculation.” Had the bombs not been used in 1945 and world leaders made aware of their frightening power, what far more awful circumstances might have unfolded in a later war when there were more nuclear powers armed with more powerful weapons? I am absolutely convinced that the bombings have had a significant deterrent effect and that they may well have prevented the outbreak of further major wars since 1945. Indeed, we know that there were occasions such as the Cuban missile crisis when national leaders looked into the maw of a possible large-scale war and backed away.
The “inoculation” issue leads to the question of whether or not America continues to need a nuclear deterrent. To this I say: “Yes, but for not entirely rational reasons.” Even very conservative military planners estimate that a few hundred warheads would be enough for any conceivable nuclear-mission scenario and that the thousands still stockpiled are a waste of resources and budgets. But the deterrent issue seems to me to be more psychological than mission-driven. With potentially unstable or irrationally-led states pursuing weapons and possibly encouraging proliferation, what “established” nuclear power would consider unilaterally disarming itself? If America and Russia engage in further rounds of treaties and draw down their numbers of deployed and reserved weapons from thousands of warheads, a time may come when these numbers will get down to those held by powers such as Britain, France, China, India and Pakistan4. How then will negotiations proceed? Even if rigorous inspection regimes are agreed to, it seems to me that it will take decades until we might get to a level of trust where we won’t feel compelled to rationalize: “They could be slipping a few weapons into their arsenal under the table; we had better keep some in reserve.” In the meantime, I encourage students and acquaintances to question their elected representatives regarding the Comprehensive Test Ban Treaty and a possible Fissile Materials Cutoff Treaty.
What about the ethics of the bombings? To my mind the answer is: “The war had rendered this issue irrelevant.” Even against the “standards” of present-day terrorist acts, the ferocity of World War II seems almost incomprehensible. Deliberate atrocities against civilians and prisoners by the Axis powers were beyond the ethical pale, but how does one classify the Allied fire-bombings of Coventry, Dresden, and Tokyo even if there were arguable military objectives? The vast majority of victims at Hiroshima and Nagasaki succumbed not to radiation poisoning but to blast and burn effects just like the victims of these other attacks. I do not see that the bombs crossed an ethical threshold that had not already been breached many times before.
What have I learned about the Manhattan Project that especially surprised me? Well, practically everything. I approached the Project as a physicist, and it was a revelation for me that much of the physics involved is entirely accessible to a good undergraduate student. Computing critical mass involves separating a spherical-coordinates differential equation and applying a boundary condition: advanced calculus. Estimating the energy released by an exploding bomb core is a nice example of using the Newtonian work-energy theorem of freshman-level physics in combination with some pressure/energy thermodynamics. Appreciating how a calutron separates isotopes is a beautiful example of using the Lorentz force law of sophomore-level electromagnetism. Estimating the chance that a bomb might detonate prematurely due to a spontaneous fission invokes basic probability theory. These are exotic circumstances which require wickedly difficult engineering to realize, but the physics is really quite fundamental.
Everybody knows that the Manhattan Project was a big undertaking, but I now realize just how truly vast it was. At first, one’s attention is drawn to the outstanding personalities and dramatic events and locales associated the Project: J. Robert Oppenheimer, Enrico Fermi, Groves, Los Alamos, Trinity, Tinian, Hiroshima and Nagasaki. Then the appreciation of the complexity of the production factories at Oak Ridge and Hanford, facilities designed by unappreciated and now largely-forgotten engineers of outstanding talent. Hundreds of contractors and university and government laboratories were involved, staffed by hundreds of thousands of dedicated employees. Also, bombs are not transported by magic to their targets; bombers had to be modified to carry them, and training of crews to fly the missions was initiated well before the final designs of the bombs and choice of targets were settled. The magnitude of the feed materials program to source and process uranium ores is rarely mentioned, but without this there would never have been any bombs (or any later Cold War).
While physics, chemistry, and engineering were front-and-center, I have also come to appreciate that the organization and administration of the Project was equally important. This is a hard thing for an academic scientist to admit! The Project was incredibly well-administered, and there is a lesson here for current times. Yes, the Project had its share of oversight and consultative committees, but they were run by scientists, engineers, government officials and military officers of superb competence and selfless dedication to the national good. These people knew what they were doing and knew how to get things done through the bureaucratic channels involved. An existential threat is always good for getting attention focused on a problem, but somebody has to actually do something. Of course there were security leaks and some inefficiencies, but what else would you expect in an undertaking so large and novel?
Could a Manhattan-type project be done now? I do not doubt for a moment that American scientists, technicians, engineers, and workers still possess the education, brains, dedication, and creativity that characterized Manhattan. But I do not think that such success could be repeated. Rather, headlines and breathless breaking news reports would trumpet waste, inefficiency, disorganization, technically clueless managers, and publicity-seeking politicians. The result would likely be a flawed product which ran far over-budget and delivered late if at all, no matter how intense the motivation. Do the words “Yucca Mountain” require further elaboration?
General Groves’ official history of the Project, the Manhattan District History, can be downloaded from a Department of Energy website, and I encourage readers to look at it5. It is literally thousands of pages, and is simply overwhelming; I doubt that anybody has read it from end-to-end. Click on any page and you will find some gem of information. Beyond the MDH lie thousands of secondary sources: books, popular and technical articles, websites and videos. But I have not one iota of regret that I plunged in. The Project was vast: many aspects of it have yet to be mined, and there are lessons to be had for scientists, engineers, biographers, historians, administrators, sociologists, and policy experts alike.
My research on the Project has made me much more aware of the world nuclear situation. Belief in deterrence aside, I am astonished that there has not been an accidental or intentional aggressive nuclear detonation over the last seventy years. We now know that on many occasions we came very close and that we have been very lucky indeed. While I see the chance of a deliberate nuclear-power-against-nuclear-power exchange as remote, the prospect of a terrorist-sponsored nuclear event does cause me no small amount of concern.
Nuclear energy is the quintessential double-edged sword, and those of us who have some understanding of the history, technicalities and current status of nuclear issues have a responsibility to share our knowledge with our fellow citizens in a thoughtful, responsible way. The stakes are no less existential now than they were seventy years ago.
Nuclear Power and Nanomaterials: Big Potential for Small Particles
Nuclear power plants are large, complex, and expensive facilities. They provide approximately 19 percent of U.S. electricity power supply,1 and in the process consume enormous quantities of water. However, a class of very small particles may be gearing up to lend a helping hand in making power plants more efficient and less costly to operate. This article will briefly introduce nanomaterials and discuss ways in which some of these particles may make nuclear power plants more efficient.
The race to synthesize, engineer, test, and apply new nanoscale materials for solving difficult problems in energy and defense is in full swing. The past twenty five years have ushered in an era of nanomaterials and nanoparticles – objects with at least one dimension between 1 and 100 nanometers2 – and researchers are now implementing these materials in areas as disparate as neuroscience and environmental remediation. To provide a sense of scale, most viruses are a few hundred nanometers in size, most bacteria are a few thousand nanometers in size, and a period at the end of a sentence is about a million nanometers. This new category of materials has ignited the imaginations of scientists and engineers who envision nanomaterials capable of tackling difficult problems in energy, healthcare, and electronics.
Nanomaterials are not new, and indeed occur naturally all over Earth. This includes viruses, the coatings of a lotus leaf, the bottom of a gecko’s foot, and some finely powdered clays. These objects represent natural materials with significant, and often highly functional, nanoscale features. Some researchers have even discovered signs of nanoscale materials in space.3 One of the oldest documented applications of nanomaterials dates back to the Lycurgus Cup, a 4th century Roman glass which was made out of a glass containing gold and silver particles. The result is a glass that appears green when lit from the outside, but red when lit from the inside.4 The effect results from the glass filtering various wavelengths of light differently depending on the various lighting conditions. Of course, the Romans did not know they were using nanoparticles in the process of making this glass.
But what makes nanoparticles interesting or unique? The answer to this question depends on the specific material and application, but a few themes persist. Because of their small size, the physical principles governing how particles behave and interact with their environments change. Some of these changes are due to how basic properties such as volume and surface area change as an object becomes smaller. As a sphere shrinks, the ratio of the surface area to the volume grows. This has far reaching implications for how particles interact with light, heat, and other particles. Visionary researchers are now looking into ways in which these interesting properties may make nuclear power plants more efficient.
One important implication for our discussion is the flow of thermal energy. Consider the process of transferring the thermal energy of your body from your hands to an ice cube. Clearly, you are (hopefully!) warmer than the ice cube. If you place the ice cube on a chilled dish and touch the ice cube with one finger, the cube will melt, but probably fairly slowly. Placing your entire hand over the top half of the ice cube increases the melting rate, and placing the ice cube in your hand and closing your fist further increases the melting rate. This is an example of thermal energy transfer via conduction. Conductive heat transfer from one object to another depends on the area over which the thermal transfer takes place. A larger contact surface area leads to faster conduction. But how does this relate to nanoparticles? As a particle becomes very small, the ratio of the particle’s surface area to its volume increases very rapidly. Since thermal conduction through volume is a function of surface area, particles with large ratios of surface area to volume are able to change temperature very quickly. If you place a large quantity of small cold particles in a warm body of water, the particles will heat quickly. If you take the same volume of particles, but instead compress it into one large particle, then that large particle will warm slowly. As this surface area to volume ratio increases with decreasing size, a general trend is for smaller particles to transfer heat more effectively than larger particles.
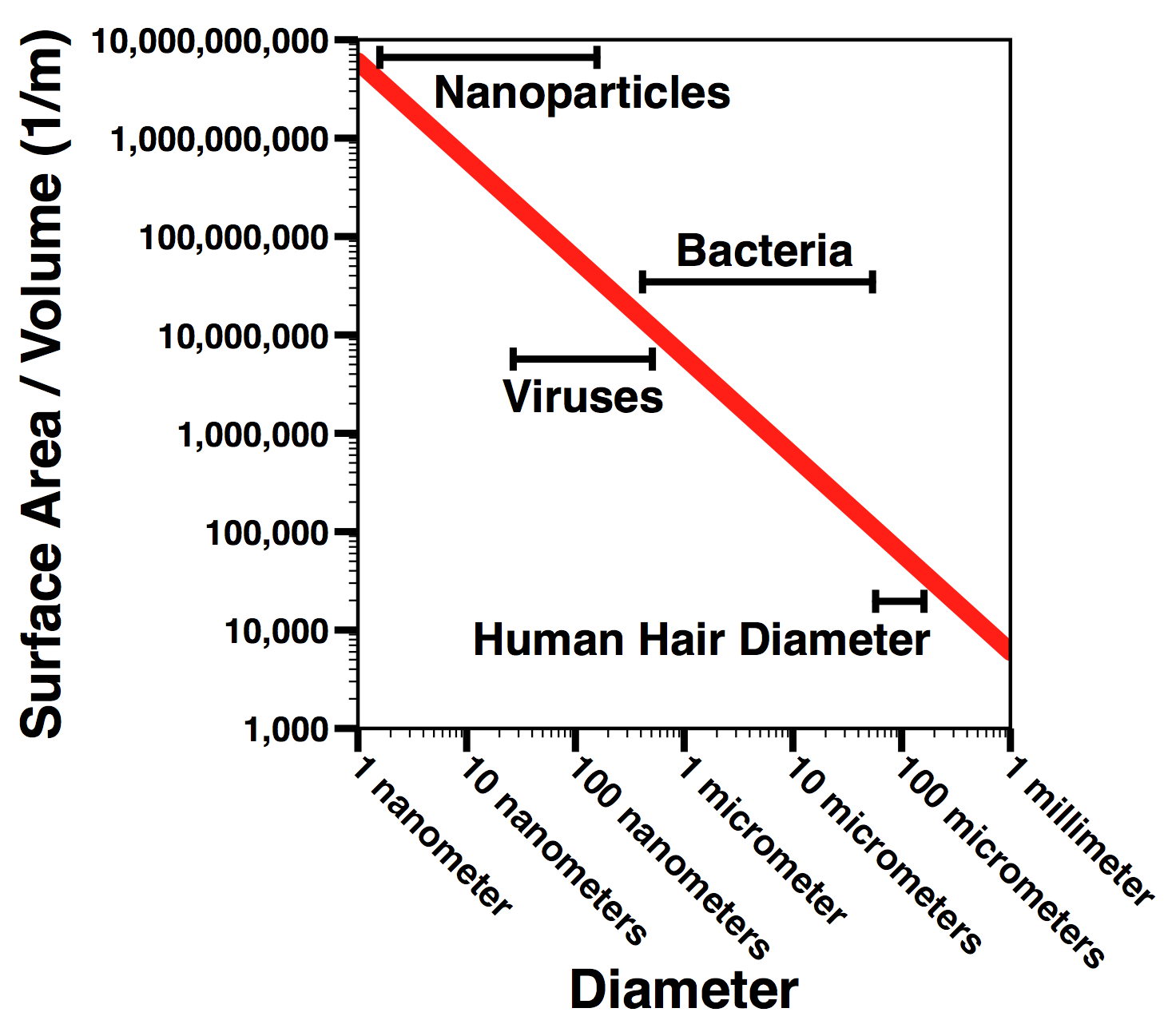
So how does this relate to nuclear power plants? Nuclear power plants are water-intense operations and rely on conductive heat transfer to convert nuclear energy to grid-ready electricity. The most common Western reactors are pressurized water reactors (PWRs) in which water is heated by pumping it through the reactor core, then pumping the hot water to a steam generator. This water flows through piping called the primary system and is kept in the liquid state by applying very high pressure through a device called a pressurizer. In the steam generator this primary system water transfers much of its heat to water in a secondary system. High-strength piping, which is a very effective heat conductor, keeps the water in the two systems from directly contacting each other. The secondary system’s water turns to steam when it absorbs the heat from the primary system. The steam is then directed via piping to drive a turbine, which turns an electric generator, thus completing the cycle of converting nuclear energy to readily usable electricity for the grid. After passing through the turbines, the steam is captured and condensed for recycling. This reclaimed water can then be sent back through the steam generator. However, a significant amount of the energy of this steam is lost to the atmosphere via a third system of cooling water that is used to condense the steam. Large amounts of water (in the form of water vapor) are released to the environment in this process. Think of the water vapor plume at the top of the iconic cooling towers seen in the cartoon TV show The Simpsons. (Not all nuclear power plants use these types of cooling towers, but all must emit heat to the environment through some means of cooling.)
A new class of nanomaterials called core-shell phase change nanoparticles may help in reducing the water loss. First, let’s parse the name of the nanoparticle. The core-shell nomenclature refers to the fact that the particle has a center made out of one material, and an outer skin made out of another material. The phase change component of the name refers to the fact that the particle center changes from a liquid to a solid under certain conditions. These particles may be mixed into the water used for transporting the thermal energy generated within the reactor. Once mixed into the reactor water, the particle cores melt as the water picks up thermal energy from the reactor. The melted material in the particle core is contained by a shell, which remains solid at reactor temperatures. Thus, as the water leaves the reactor it carries with it tiny particles containing bundles of liquid thermal energy wrapped in a solid core. The notion is that as these particles travel to the cooling tower, they solidify and dissipate their heat into the surrounding water, thus decreasing the amount of water needed to convert the thermal energy created by the reactor to steam for turning turbines. Additionally, since these particles do not vaporize, they are much more easily retained for recycling. The Electric Power Research Institute is currently working with scientists at Argonne National Laboratory to commercialize these particles and has suggested that this technology could decrease power plant water requirements by as much as 20 percent.5
Another nanoparticle-based approach for increasing reactor efficiency seeks to tackle a different problem. Pressurized water reactors place the water in direct contact with the fuel rods of the nuclear reactor. However, bubbles that form on the surfaces of the fuel rods can significantly decrease efficiency by insulating the rods from the water. When this happens, heat transfer efficiency suffers. One lab at the Massachusetts Institute of Technology (MIT) has implemented alumina nanoparticles that coat the fuel rods and prevent the buildup of bubbles on the heating elements. Alumina, a compound of aluminum and oxygen, is stable and has a high melting temperature. Testing these particles in the MIT reactor, the group found that the alumina nanoparticles coated the fuel rods. The result was an increase in the efficacy of the reactor. The engineers explain the findings by suggesting that the alumina nanoparticles allow for quick removal of the bubbles forming on fuel rod surfaces, thus minimizing the insulating layer of bubbles and maximizing heat transfer efficiency.6 To validate this, the researchers heated identical thin, steel wires a fraction of a millimeter in diameter. One wire was submerged in water, the other in a nanofluid containing alumina particles. The wires were heated to the point of boiling the surrounding fluid. After boiling the wires were examined using a powerful electron microscope. The experimenters observed that the wire heated in the nanofluid was indeed coated with nanoparticles, while the other wire maintained its original smooth surface.
Most importantly, there are also potential safety applications of having nanofluids capable of quickly transporting large quantities of thermal energy. One proposal calls for the use of nanofluids in standby coolant stored in Emergency Core Cooling Systems (ECCS). The ECCS are independent, standby systems designed to safely shut down a reactor in the case of an accident or malfunction. One ECCS component is a set of pumps and backup coolant to be sprayed directly onto reactor rods. Such systems are critical in preventing a loss of coolant accident (LOCA) from spiraling out of control. Because ECCS have backup reservoirs of coolant, technologies that make this backup coolant more effective at removing heat from the reactor could improve the safety of reactors. Because nanofluids can increase the heat transfer efficacy of water by 50 percent or more, some researchers have suggested that they may also be useful in emergency scenarios.7
Steam generators at both nuclear and coal power plants accounts for approximately 3 percent of overall freshwater consumption in the United States. Generally speaking, nuclear power plants consume about 400 gallons of water per megawatt-hour (MWh). Their coal and natural gas counterparts consume approximately 300 and 100 gallons per MWh, respectively.8 Thus, nuclear power plants stand to gain considerably by becoming more water-efficient.
However, there are many hurdles to tackle before nanoparticles can be safely and effectively used in operating power plants. Scaling up particle production to the large volumes of particles necessary for implementation in a power plant is expensive and labor intensive. New synthesis infrastructures may be necessary for large-scale production of these tiny particles. Additionally, broad adoption of this technology will not occur until significant cost savings are proven effective at a functioning plant. As a result, particles must be made available at a cost reasonable for adoption by power plant operators. A rough cost estimate can be made using commercially available alumina nanoparticles, as these particles have been tested extensively in the heat transfer literature. A typical nuclear power plant in the United States supplies enough electricity to power 740,000 homes. To do this, the plant requires between 13 and 23 gallons of water per home per day. Thus, water usages for the plant may range from 10 to 17 million gallons per day. Current vendors of aluminum oxide nanoparticles sell 1kg of nanopowder for around $200. With an expectation that economies of scale would bring that price down to $100/kg and that the particles could be easily recovered and recycled, loading a nuclear power plant with a 0.1% volume fraction of alumina nanoparticles would cost about $14.7 to $25 million per power plant. This is a substantial initial investment. Naturally, if nanoparticles were to cost $10/kg, then particle outfitting costs of $1.5 to $2.5 million per nuclear plant could be achieved. If 100 percent recovery of the particles could be achieved, then this initial cost would be recovered over time by the expected 2 to 4 percent increase in plant efficiency.
In addition to cost-benefit analysis, extensive testing must be performed to ensure long-term application of these particles does not threaten the operational safety of the plant. To accomplish this, smaller scale reactors (like those housed at research facilities and universities) may test these particles over the course of years to track the impacts of long-term use. Potential pitfalls include increased corrosion, system clogging, and nanoparticle leakage into wastewater. Corrosion engineers will be needed to validate the degree to which nanoparticles contribute to the overall aging of reactors in which they are used. Nanoparticle designers and hydrodynamicists will be needed to ensure that system clogging is manageable. Additionally, filtration experts and the Environmental Protection Agency will be needed to establish best practices for minimizing the amount of nanomaterial that exits the facility, as well as understanding and quantifying the environmental impacts of that emitted material. None of these potential roadblocks are trivial. However, while the challenges seem large, it is encouraging to see potential applications of nanotechnology in power plants.
The Making of the Manhattan Project Park
The making of the Manhattan Project National Historical Park took more than five times as long as the making of the atomic bomb itself (1942 to 1945). Fifteen years after the first efforts to preserve some of the Manhattan Project properties at Los Alamos, New Mexico, in 1999, Congress enacted the Manhattan Project National Historical Park Act, signed by President Obama on December 19, 2014. The following provides the story of how the park was created and a preview of coming attractions.
Mandate for a Clean Sweep
After the end of the Cold War in 1989, Congress directed the Department of Energy (DOE) to clean up decades of contamination at its nuclear production facilities. At Los Alamos, the V Site (where the atomic bombs were assembled), was a cluster of garage-like wooden structures left over from the Manhattan Project, far from public view. The main property had high-bay doors to accommodate the “Gadget,” the world’s first atomic device tested at the Trinity Site on July 16, 1945. Along with dozens of other Manhattan Project properties, the Los Alamos National Laboratory (LANL) slated the V Site buildings for demolition.
LANL officials estimated that the costs just to stabilize the buildings would be $3 million. “Preservation would be a waste of taxpayers’ money1,” declared LANL’s Richard Berwick. When the State of New Mexico concurred in the demolition, the buildings were doomed.
Rescuing the V Site Properties
The legacy of the Manhattan Project was in the crosshairs. Were any of the original Manhattan Project properties at Los Alamos going to be saved? Working for the Department of Energy, I called the Advisory Council on Historic Preservation (ACHP) for advice. The Council agreed to add a day to its Santa Fe meeting that fall to visit the V Site.
On November 5, 1998, the Advisory Council members were astonished by the contrast between the simplicity of V Site properties and the complexity of what took place inside them. The group concluded that the V Site would not only qualify as a National Historic Landmark but as a World Heritage Site similar to the Acropolis in Athens or the ancient city of Petra in Jordan. Somewhat chastened, the Los Alamos National Laboratory agreed to take the cluster of V Site buildings off the demolition list. However, funds to restore them would have to come from elsewhere.
Save America’s Treasures
In 1998 Congress and First Lady Hillary Clinton decided to commemorate the millennium by awarding Save America’s Treasures grants to preserve historic federal properties in danger of being lost. In a competitive process run by the National Park Service, the Department of Energy (DOE) was awarded $700,000 to restore the V Site properties.
However, there was a catch-22: the grant had to be matched by non-federal funds, but federal employees cannot solicit funds and DOE has no foundation authorized to do so. Rather than have DOE forfeit the grant, I decided to leave a 25-year career with the federal government in January 2000 to raise the funds and segue to my next “real” job.
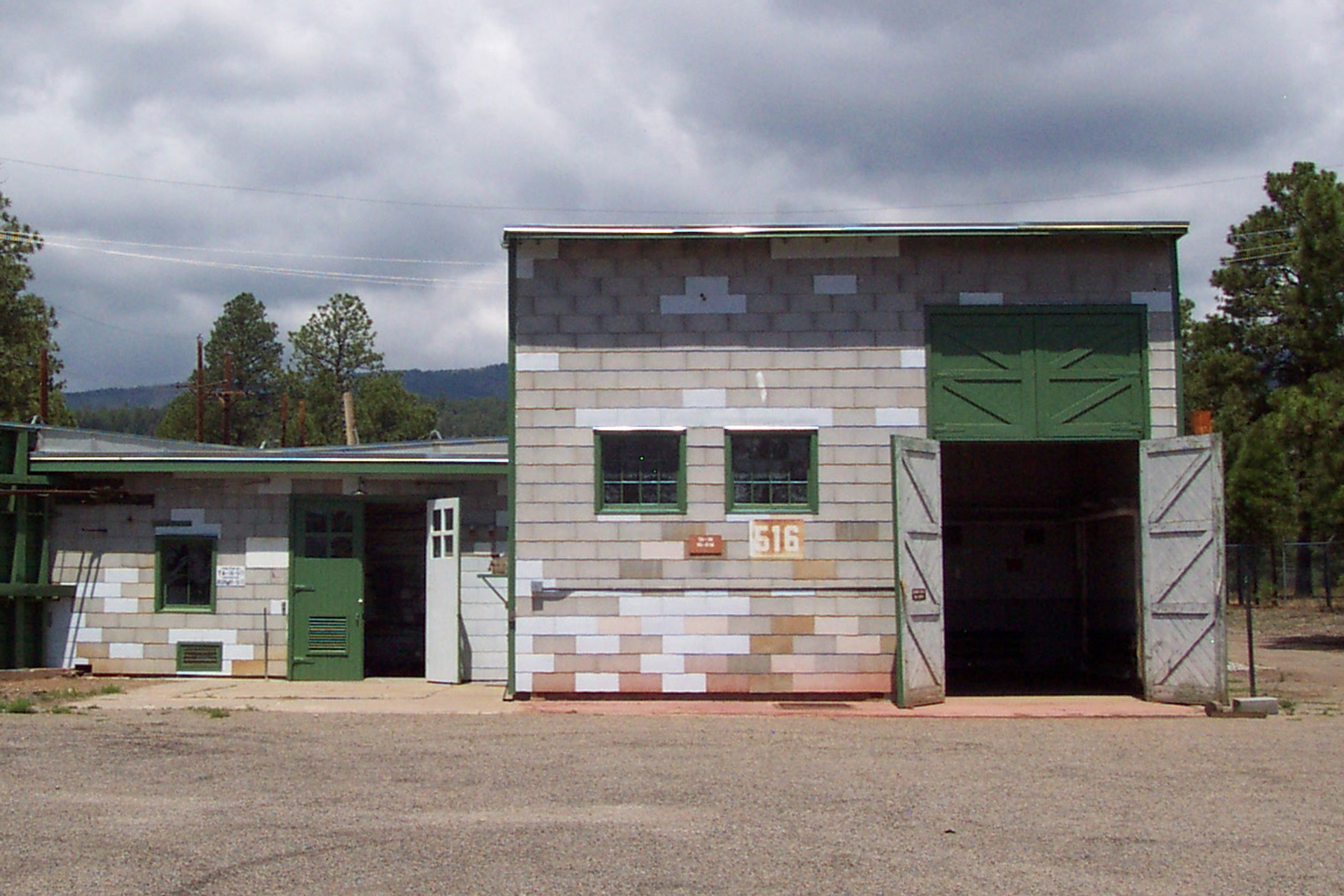
Restored V Site at Los Alamos
Gaining Traction
The fund-raising project quickly evolved into a much bigger effort. To galvanize public and political attention, in March 2001 I enlisted the Los Alamos Historical Society to collaborate on a weekend of events called “Remembering the Manhattan Project.” The centerpiece was the “Louis Slotin Sonata,” a new play by Paul Mullin about a Manhattan Project scientist who died in a criticality experiment at Los Alamos in early 1946. The play and a heated discussion afterwards was covered by the New York Times and other press, bringing the Manhattan Project to national attention.
In February 2002, I founded the Atomic Heritage Foundation (AHF), a nonprofit in Washington, DC dedicated to preserving and interpreting the Manhattan Project. Richard Rhodes, Pulitzer Prize-winning author of The Making of the Atomic Bomb, helped open doors to Senators Jeff Bingaman (D-NM), and Pete Domenici (R-NM). To increase interest in preserving the Manhattan Project, in April 2002 we convened a symposium in Washington, DC that was covered by C-SPAN worldwide.
On September 30, 2003, Senators Bingaman, Maria Cantwell (D-WA), and Patty Murray (D-WA), introduced legislation to study the potential for including the Manhattan Project in the National Park System. On the same day, Congressman Doc Hastings (R-WA), introduced similar legislation in the House. Congress passed the study bill in the fall of 2004 and President George W. Bush signed it despite the administration’s opposition to any new parks.
For more than a decade, the Congressional delegations from New Mexico, Washington and Tennessee were a very strong, bipartisan team. Their commitment to the park was critical at every juncture over the next decade but especially in the final weeks of the Congress. The last major public lands omnibus legislation was in 2009; since then very few park bills had been passed. The Senate had a long list of bills that it wanted to attach to the NDAA along with the Manhattan Project National Historical Park. However, efforts to create a small “package” of other bills failed in 2013. Finally, in December 2014, the House passed the legislation as part of the “must pass” 2015 National Defense Authorization Act.
Attaching a large public lands “package” was risky as there was strong opposition in the Senate to expanding public lands and creating new parks. With several close calls in the days before its passage, this time the strategy succeeded. Congress passed the NDAA with a robust “package” of six new national park units, nine park expansions and dozens of other public lands provisions. On December 19, 2014, the President signed the legislation into law.
The new Manhattan Project National Historical Park has units at Los Alamos, NM, Oak Ridge, TN, and Hanford, WA. During World War II, these “secret cities” were not on any map even though some 130,000 people lived in them.
The park will be officially established in late 2015 when the Departments of Energy and Interior enter into an agreement concerning their respective roles, public access and other issues.
Preview of the Park
The new park will focus on three major sites: Los Alamos, NM, where the first atomic bombs were designed; Oak Ridge, TN, where enormous facilities produced enriched uranium; and Hanford, WA, where plutonium was produced. There are over 40 properties that are officially designated as part of the park with provision for adding others later.
Los Alamos, NM
The new park includes 13 properties in the Los Alamos community, many of them originally built by the Los Alamos Ranch School in the 1920s. The government took over the school’s properties in 1943 for the Manhattan Project. The seven former Masters’ cottages became the homes of the top-echelon scientists and military leaders. Because these cottages were the only housing with bathtubs, the street became known as Bathtub Row.
The cottage where J. Robert Oppenheimer and his family lived could be the “jewel in the crown” of the visitors’ experience. Visitors are also welcome at the Guest House, now the Los Alamos Historical Society Museum, and the Fuller Lodge, a handsome ponderosa pine structure that was a social center for the Manhattan Project.
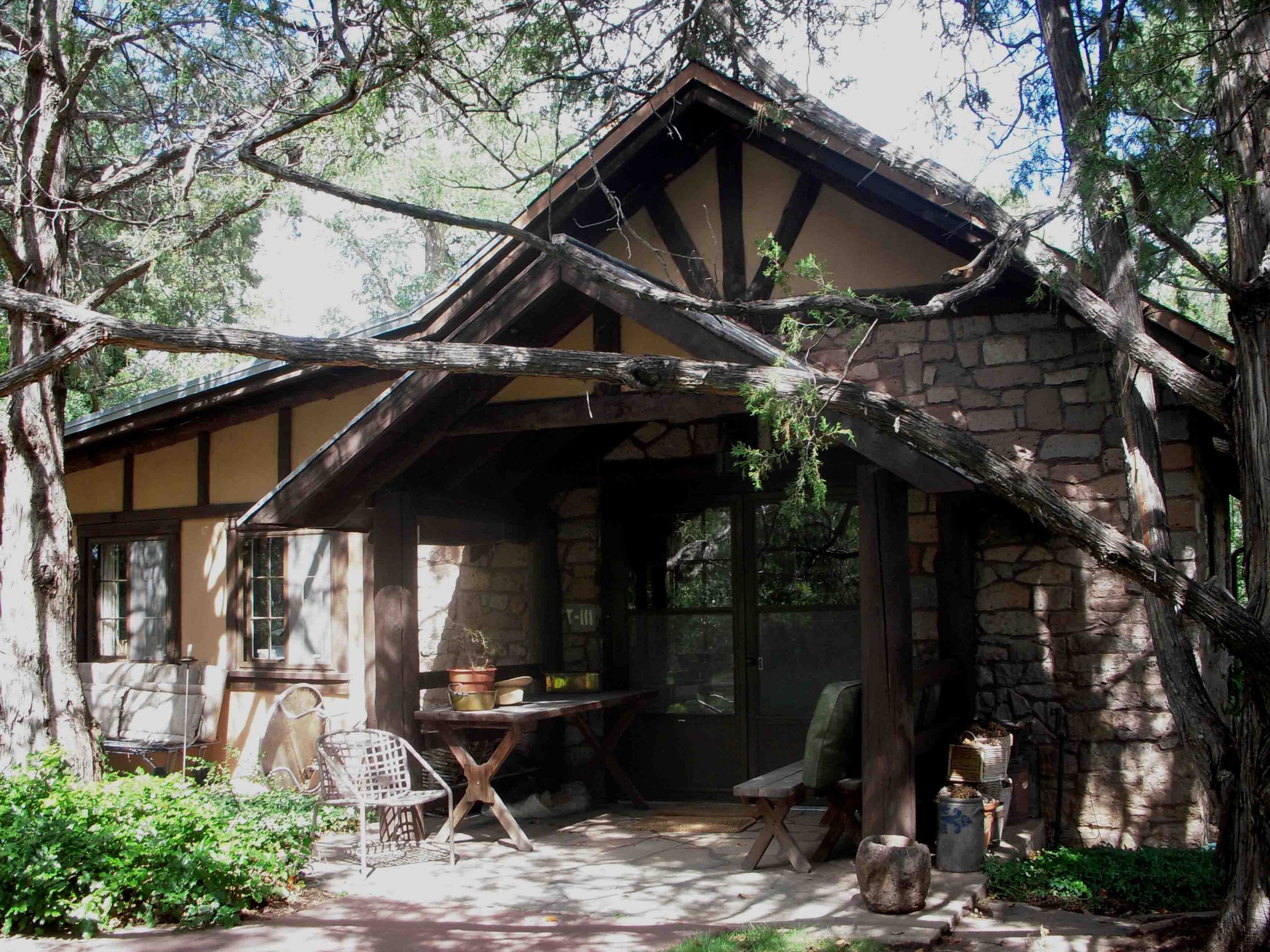
Oppenheimer House, Los Alamos
More than a dozen other properties are owned by the Los Alamos National Laboratory. Public access to these properties could be limited for the first few years to address security issues. The V-Site buildings, saved from demolition in 1998 and restored in 2006, are humble garage-like structures were where the “Gadget” was assembled. The “Gadget” was the initial plutonium-based bomb that was tested at the Trinity Site on July 16, 1945.
A companion facility to the V Site is the Gun Site used to develop and test the “Little Boy” or uranium-based bomb. The gun-type design fired a small projectile of uranium into a greater mass to create an explosion. The Gun Site is undergoing reconstruction but will eventually have a concrete bunker, periscope tower, canons and a firing range.
Oak Ridge, TN
The mission of the Clinton Engineer Works was to produce enriched uranium, one the core ingredients of an atomic bomb. Mammoth plants at Y-12 and K-25 used different techniques to produce enriched uranium. While security is an issue now, visitors will eventually be able to tour the remaining “Calutron” building at Y-12. While the mile-long K-25 building was demolished last year, plans are to recreate a portion of it for visitors.
A third site at Oak Ridge is the X-10 Graphite Reactor, a pilot-scale reactor and prototype for the Hanford plutonium production reactors. Visitors will be able to see the former Guest House (later named the Alexander Inn) built to accommodate distinguished visitors such as General Leslie Groves, Enrico Fermi, and Ernest O. Lawrence. Recently restored as a residence for seniors, the lobby will have Manhattan Project photographs and other memorabilia.
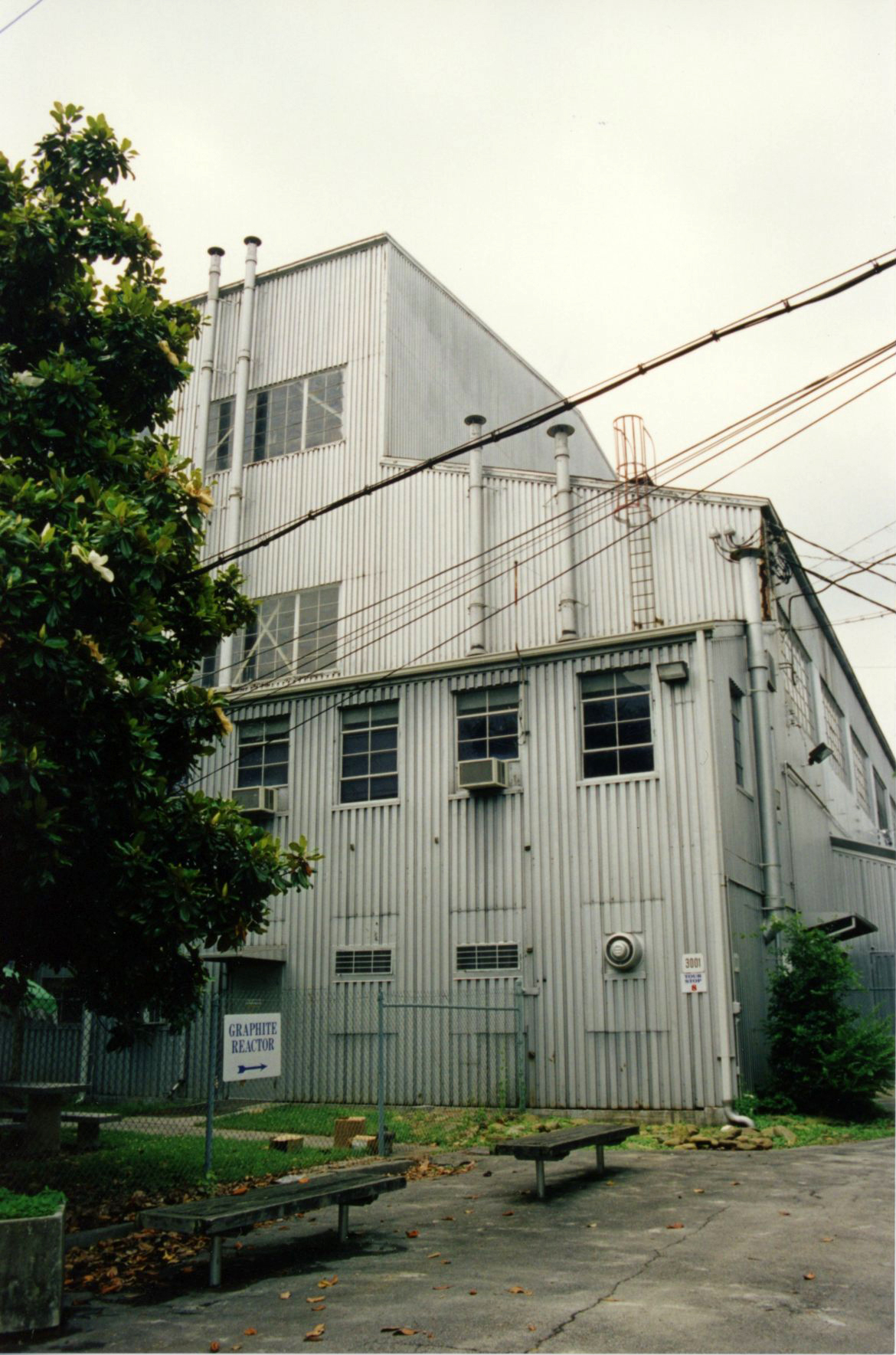
X-10 Site, Oak Ridge
Hanford, WA
There are two iconic Manhattan Project properties at Hanford. The B Reactor, the world’s first full-scale plutonium production reactor, has been welcoming visitors for several years. There many interpretive displays and models that the Atomic Heritage and B Reactor Museum Association have developed. For example, there is an interactive model of the B reactor and the dozens of support buildings that once surrounded it. There is also a cutaway model of the reactor core showing the lattice of uranium fuel rods, graphite blocks, control rods and other features.
The second property is the T Plant, a mammoth “Queen Mary” of the desert used to chemically separate plutonium from irradiated fuel rods. It was one of the first remotely controlled industrial operations. Prospects are that the public will be able to visit a portion of the plant over time.
In addition, four pre-World War II properties located along the Columbia River will be preserved: the Hanford high school, White Bluffs bank, an agricultural warehouse owned by the Bruggemann family, and an irrigation pump house. Here visitors will hear the stories of the pioneering agricultural families as well as the Native Americans who lived, hunted and fished and camped near the Columbia River.
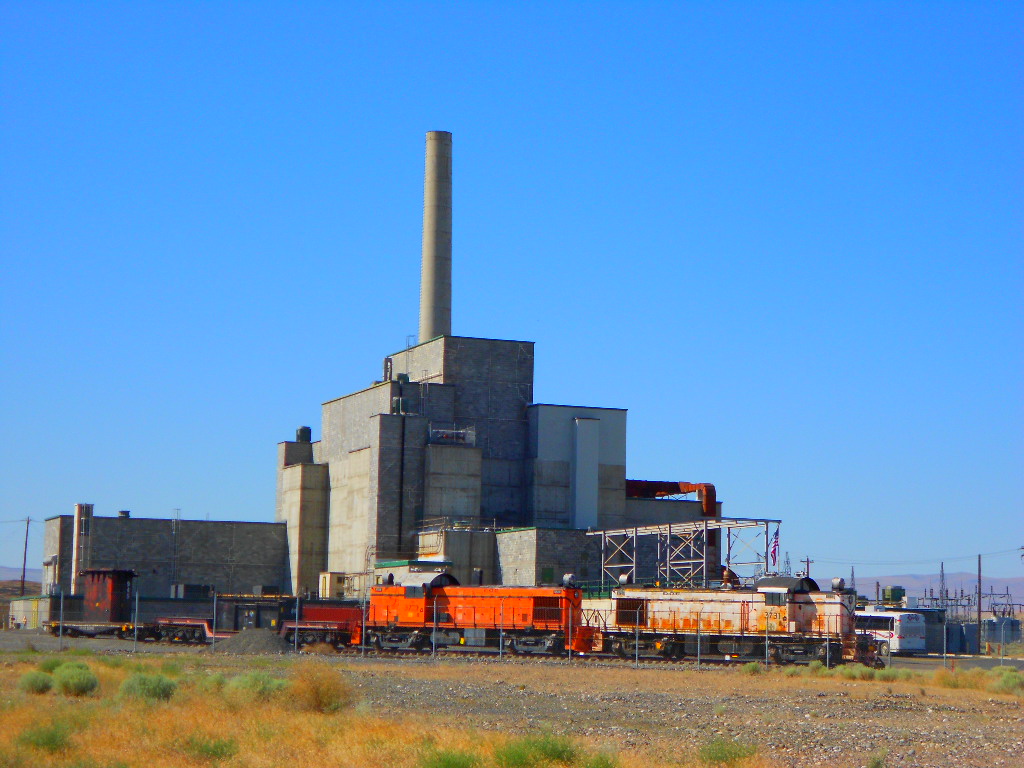
B Reactor, Hanford
At each site, visitors will be able to experience where people lived—in tents, huts, trailers, barracks, and dormitories or for the lucky ones, houses. In the communities of Richland, WA and Oak Ridge, TN, hundreds of “Alphabet” houses built from the same blueprints have been home for families for over seven decades.
For the Atomic Heritage Foundation2, the creation of the Manhattan Project National Historical Park is the culmination of 15 years of effort. Like the Manhattan Project itself, creating a national historical park has been a great collaborative effort.
Perhaps the greatest source of inspiration has been the Manhattan Project veterans themselves. To Stephane Groueff, a Bulgarian journalist who wrote the first comprehensive account of the Manhattan Project3 the participants illustrated “the American way of the time…problem solving, ingenuity, readiness for risk-taking, courage for unorthodox approaches, serendipity, and dogged determination4.” There are many lessons that we can learn from the Manhattan Project.
Please join us for a symposium to mark the 70th anniversary of the Manhattan Project on June 2 and 3, 2015 in Washington, DC. Also, please visit our “Voices of the Manhattan Project5” website with hundreds of oral histories including of principals such as General Leslie Groves and J. Robert Oppenheimer. Our “Ranger in Your Pocket6” website has a series of audio/visual tours of the Manhattan Project sites that visitors can access on their smartphones and tablets. Most of all, plan on visiting the Manhattan Project National Historical Park. Coming soon!
Public Interest Report: February 2015
Seeking China-U.S. Strategic Nuclear Stability
by Charles D. Ferguson
Can NFU be demonstrated? Some analysts have argued that China in its practice of keeping warheads de-mated or unattached from the missile delivery systems has in effect placed itself in a second strike posture. But the worry from the American side is that such a posture could change quickly and that as China has been modernizing its missile force from slow firing liquid-fueled rockets to quick firing solid-fueled rockets, it will be capable of shifting to a first-use policy if the security conditions dictate such a change.
Look to Texas Rather Than Nevada for a Site Selection Process on Nuclear Waste Disposal
by Daniel Sherman
Nevada’s persistent and successful efforts to thwart the Yucca Mountain project and the Nuclear Waste Policy Act of 1982 are likely to continue as they demonstrate the futility of a policy that forces disposal on an unwilling host state.
Reflections on the 70th Anniversary of the Manhattan Project: Questions and Answers
by B. Cameron Reed
Could a Manhattan-type project be done now? I do not doubt for a moment that American scientists, technicians, engineers, and workers still possess the education, brains, dedication, and creativity that characterized Manhattan. But I do not think that such success could be repeated.
Nuclear Power and Nanomaterials: Big Potential for Small Particles
by Lamar O. Mair
The race to synthesize, engineer, test, and apply new nanoscale materials for solving difficult problems in energy and defense is in full swing. The past twenty five years have ushered in an era of nanomaterials and nanoparticles – objects with at least one dimension between 1 and 100 nanometers.
The Making of the Manhattan Project Park
by Cynthia C. Kelly
The making of the Manhattan Project National Historical Park took more than five times as long as the making of the atomic bomb itself.
Public Interest Report: November 2014
Energy Policy and National Security: The Need for a Nonpartisan Plan
by Charles D. Ferguson
Once the global decline starts to take effect, price shocks could devastate the world’s economy. Moreover, as the world’s population is projected to increase from seven billion people today to about nine billion by mid-century, the demand for oil will also significantly increase given business as usual practices.
Is ISIL a Radioactive Threat?
by George M. Moore
Is there a real potential that ISIL could produce a “dirty bomb” and inflict radiation casualties and property damage in the United States, Europe, or any other state that might oppose ISIL as part of the recently formed U.S.-led coalition?
Thinking More Clearly About Nuclear Weapons: The Ukrainian Crisis’ Overlooked Nuclear Risk
by Martin Hellman
It is surprising and worrisome that almost none of the mainstream media’s coverage of the Ukrainian crisis has mentioned its nuclear risk. With the West feeling that Russia is solely to blame, and Russia having the mirror image perspective, neither side is likely to back down if one of their red lines is crossed.
A Looming Crisis of Confidence in Japan’s Nuclear Intentions
by Ryan Shaffer
Global nonproliferation principles undoubtedly remain a high priority for Japan. But it is likely that in the short term, the eyes of Japan’s leaders are focused more intently on bringing nuclear reactors back on line.
The CTBT: At the Intersection of Science and Politics
by Jenifer Mackby
Although the treaty deals with the highly technical and sensitive subject of nuclear test explosions, it has been considered in a political context since the negotiations. Countries possessing nuclear weapons do not want others to know about their facilities or capabilities, so verification provisions of the treaty were exceptionally difficult to negotiate.
Seismic Risk Management Solution for Nuclear Power Plants
by Justin Coleman and Piyush Sabharwall
Management of external hazards to expectable levels of risk is critical to maintaining nuclear facility and nuclear power plant safety.
Is ISIL a Radioactive Threat?
In the past several months, various news stories have raised the possibility that the Islamic State of Iraq and the Levant (ISIL, also commonly referred to as ISIS) could pose a radioactive threat. Headlines such as “Dirty bomb fears after ISIS rebels seize uranium stash,”1 “Stolen uranium compounds not only dirty bomb ingredients within ISIS’ grasp, say experts,”2 “Iraq rebels ‘seize nuclear materials,’” 3 and “U.S. fears ISIL smuggling nuclear and radioactive materials: ISIL could take control of radioactive, radiological materials”4 have appeared in mainstream media publications and on various blog posts. Often these articles contain unrelated file photos with radioactive themes that are apparently added to catch the eye of a potential reader and/or raise their level of concern.
Is there a serious threat or are these headlines over-hyped? Is there a real potential that ISIL could produce a “dirty bomb” and inflict radiation casualties and property damage in the United States, Europe, or any other state that might oppose ISIL as part of the recently formed U.S.-led coalition? What are the confirmed facts? What are reasonable assumptions about the situation in ISIL-controlled areas and what is a realistic assessment of the level of possible threat?
As anyone who has followed recent news reports about the rapid disintegration of the Iraqi Army in Western Iraq can appreciate, ISIL is now in control of sizable portions of Iraq and Syria. These ISIL-controlled areas include oilfields, hospitals, universities, and industrial facilities, which may be locations where various types of radioactive materials have been used, or are being used.
In July 2014, the International Atomic Energy Agency (IAEA) released a statement indicating that Iraq had notified the United Nations “that nuclear material has been seized from Mosul University.”5 The IAEA’s press release indicated that they believed that the material involved was “low-grade and would not present a significant safety, security or nuclear proliferation risk.” However, despite assessing the risk posed by the material as being low, the IAEA stated that “any loss of regulatory control over nuclear and other radioactive materials is a cause for concern.”6 The IAEA’s statement caused an initial flurry of press reports shortly after its release in July.
A second round of reports on the threat of ISIL using nuclear or radioactive material started in early September, triggered by the announcement of a U.S.-Iraq agreement on a Joint Action Plan to combat nuclear and radioactive smuggling.7 According to a Department of State (DOS) press release on the Joint Action Plan, the U.S. will provide Iraq with training and equipment via the Department of Energy’s Global Threat Reduction Initiative (GTRI) that will enhance Iraq’s capability to “locate, identify, characterize, and recover orphaned or disused radioactive sources in Iraq thereby reducing the risk of terrorists acquiring these dangerous materials.”8 Although State’s press release is not alarmist, it does state that the U.S. and Iraq share a conviction that nuclear smuggling and radiological terrorism are “critical and ongoing” threats and that the issues must be urgently addressed.9
While September’s headlines extrapolating State’s press release to U. S. “fears” might be characterized by some as over-hyping the issue, it is clear that both statements from the IAEA and the State Department have indicated that the situation in Iraq may be cause for concern. Did IAEA and DOS go too far in their statements? In their defense, it would be highly irresponsible to indicate that any situation where nuclear or other radioactive material might be in the hands of individuals or groups with a potential for criminal use is not a subject for concern. However, we need to go beyond such statements and determine what risks are posed by the materials that have been reported as possibly being under ISIL control in order to determine how concerned the public should be.
According to the IAEA’s press release, the material reported by Iraq was described as “nuclear material,” but this description does not imply that it is suitable for a yield producing nuclear weapon. In fact, the IAEA’s description of the material as “low-grade” indicates that the IAEA believes that this material is not enriched to the point where it could be used to produce a nuclear explosion. Furthermore, although the agency has not provided a technical description of the nuclear material, it is highly unlikely that this is anything other than low enriched uranium or perhaps even natural or depleted uranium, all of which would fit under the IAEA’s definition of “nuclear material.” If the material is not useful in a yield producing device, is it a radioactive hazard? All forms of uranium are slightly radioactive, but the level of radioactivity is so low that these materials would not pose a serious radioactive threat, (either to persons or property), if they were used in a Radioactive Dispersion Devices (RDDs). Even a “Dirty Bomb,” which is an RDD dispersed by explosives, would not be of significant concern.
Other than the nuclear material mentioned in the report to the United Nations, there are no known open source reports of loss of control of other radioactive materials. However, a lack of specific reporting does not mean that control is still established over any materials that are in ISIL -controlled areas. It would be prudent to assume that all materials in these areas are out of control and assessable to ISIL should it choose to use whatever radioactive materials can be found for criminal purposes. How do we know what materials may be at risk? Hopefully the Iraq Radioactive Sources Regulatory Authority (IRSRA) has/had a radioactive source registry in Iraq. If so, authorities should know in some detail what materials are in ISIL-controlled territories. The Syrian regulatory authority may have at one time had a similar registry that would indicate what may now be out of control in the ISIL-controlled areas of Syria. Unfortunately, there is no open source reporting of any of these materials so we are left to speculate as to what might be involved and what the consequences may be should those materials attempt to be used criminally.
It is doubtful that any radioactive materials in ISIL controlled areas are very large sources. The materials that would pose the greatest risk would probably be for medical uses. These sources are found in hospitals or clinics for cancer treatment or blood irradiation and typically use cesium 137 or cobalt 60, both of which are relatively long-lived (approximately 30 and five years respectively) and produce energetic gamma rays. It is also possible that radiography cameras containing iridium 192 and well logging sources that typically use cesium 137 and an americium beryllium neutron source may also be in the ISIL-controlled areas. Any technical expert would opine that these sources are capable of causing death and that dispersal of these materials would create a cleanup problem and possibly significant economic loss. However, experts almost uniformly agree that such materials do not constitute Weapons of Mass Destruction, but are potential sources for disruption and for causing public fear and panic. Furthermore the scenarios that pose the greatest risk for the United States or Europe from these materials are difficult for ISIL to organize and carry out.
If ISIL were to attempt to use such materials in an RDD, they would need to transport the materials to the target area (for example in the United States or Europe), in a manner that is undetectable and relatively safe for the person(s) transporting or accompanying a movement of the material. Although in some portions of a shipment cycle there would be no need to accompany the materials, at some point people would need to handle the materials. Even if the handlers had suicidal intent, shielding would be required in order to prevent detection of the energetic radiations that would be present for even a weak RDD. Shielding required for really dangerous amounts of these materials is typically both heavy and bulky and therefore the shielded materials cannot be easily transported simply by a person carrying them on their person or in their luggage. They would probably need to be shipped as cargo in or on some sort of vehicle (car, bus, train, ship, or plane). Surface methods of transport might reach Europe, but carriage by ship or air is necessary to reach the United States.10 Aircraft structures do not provide any inherent shielding and so the most logical (albeit not only), method of transportation to the United States or Europe would be by ship, probably from a Syrian port. Even though ISIL controls a significant land area, the logistics of shipping an item that is highly radioactive to the United States or Europe would be a complex process and need to defeat significant post-9/11 detection systems. These systems, although perhaps not 100 percent effective for all types and amounts of radioactive material, typically are thought to be very effective in the detection of high-level sources.
Any materials from ISIL-controlled areas could only be used in the United States or Europe with great difficulty. It is highly probable that the current radiation detection systems would be effective in deterring any such attempted use even if there were no human intelligence that would compromise such an effort. Even if ISIL could use materials for an RDD attack, the actual damage potential of these types of attacks is relatively low when they are compared to far simpler and often used terrorist tactics such as suicide vests and truck and car bombs. The casualties that would result from any theoretical RDD would be probably less than those resulting from a serious traffic accident and that is probably on the high end of casualty estimates. Indeed, many experts feel that most, if not all, of the serious injuries from a “dirty bomb” would result from the explosive effects of the bomb, not from the dispersal of radioactive materials. The major consequence of even a fairly effective dispersal of material would be a cleanup problem with the economic impact determined by the area contaminated and the level to which the area would need to be cleaned.
Efforts by the United States to work with the ongoing government in Iraq in improving detection and control of nuclear and other radioactive materials appear to be a prudent effort to minimize any threat from these materials in the ISIL-controlled areas. To date, ISIL has not made any threats to use radioactive material. That does not mean that ISIL is unaware of the potential, and we should be prepared for ISIL to use their surprisingly effective social media connections to attempt to make any future radioactive threat seem apocalyptic. Rational discussion of potential consequences and responses to an attack scenario should occur before an actual ISIL threat, rather than having the discussion in the 24/7 news frenzy that could invariably follow an ISIL threat.
Dr. George Moore is currently a Scientist in Residence and Adjunct Faculty Member at the Monterey Institute of International Studies’ James Martin Center for Nonproliferation Studies, a Graduate School of Middlebury College, in Monterey, California. He teaches courses and workshops in nuclear trafficking, nuclear forensics, cyber security, drones and surveillance, and various other legal and technical topics. He also manages an International Safeguards Course sponsored by the National Nuclear Security Agency in cooperation with Lawrence Livermore National Laboratory.
From 2007-2012 Dr. Moore was a Senior Analyst in the IAEA’s Office of Nuclear Security where he was involved with, among other issues, the IAEA’s Illicit Trafficking Database System and the development of the Fundamentals of Nuclear Security publication, the top-level document in the Nuclear Security Series. Dr. Moore has over 40 years of computer programming experience in various programming languages and has managed large database and document systems. He completed IAEA training in cyber security at Brandenburg University and is the first instructor to use the IAEA’s new publication NS22 Cyber Security for Nuclear Security Professional as the basis for a course.
Dr. Moore is a former staff member of Lawrence Livermore National Laboratory where he had various assignments in areas relating to nuclear physics, nuclear effects, radiation detection and measurement, nuclear threat analysis, and emergency field operations. He is also a former licensed research reactor operator (TRIGA).
Energy Policy and National Security: The Need for a Nonpartisan Plan
As I write this president’s message, the U.S. election has just resulted in a resounding victory for the Republican Party, which will have control of both the Senate and House of Representatives when the new Congress convenes in January. While some may despair that these results portend an even more divided federal government with a Democratic president and a Republican Congress, I choose to view this event as an opportunity in disguise in regards to the important and urgent issue of U.S. energy policy.
President Barack Obama has staked a major part of his presidential legacy on combating climate change. He has felt stymied by the inability to convince Congress to pass comprehensive legislation to mandate substantial reductions in greenhouse gas emissions. Instead, his administration has leveraged the power of the Environmental Protection Agency (EPA) to craft rules that will, in effect, force the closure of many of the biggest emitters: coal power plants. These new rules will likely face challenges in courts and Congress. To withstand the legal challenge, EPA lawyers are working overtime to make the rules as ironclad as possible.
The Republicans who oppose the EPA rules will have difficulty in overturning the rules with legislation because they do not have the veto-proof supermajority of two-thirds of Congress. Rather, the incoming Senate majority leader Mitch McConnell (R-Kentucky) said before the election that he would try to block appropriations that would be needed to implement the new rules. But this is a risky move because it could result in a budget battle with the White House. The United States cannot afford another grinding halt to the federal budget.
Several environmental organizations have charged many Republican politicians with being climate change deniers. Huge amounts of money were funneled to the political races on both sides of the climate change divide. On the skeptical side, political action groups affiliated with the billionaire brothers Charles and David Koch received tens of millions of dollars; they have cast doubt on the scientific studies of climate change. And on the side of wanting to combat climate change, about $100 million was committed by NextGen Climate, a political action group backed substantially by billionaire Tom Steyer. Could this money have been better spent on investments in shoring up the crumbling U.S. energy infrastructure? Instead of demonizing each side and just focusing on climate change, can the nation try a different approach that can win support from a core group of Democrats and Republicans?
Both Democratic and Republican leaders believe that the United States must have strong national security. Could this form the basis of a bipartisan plan for better energy policy? But this begs another question that would have to be addressed first: What energy policy would strengthen national security? Some politicians, including several former presidents, have called for the United States to be energy independent. Due to the recent energy revolution in technologies to extract so-called unconventional oil and gas from shale and sand geological deposits, the United States is on the verge of becoming a major exporter of natural gas and has dramatically reduced its dependence on outside oil imports (except from the friendly Canadians who are experiencing a bonanza in oil extracted from tar sands). However, these windfall developments do not mean that the United States is energy independent, even including the natural resources in all of North America.
Oil is a globally traded commodity and natural gas (especially in the form of liquefied natural gas) is tending to become this type of commodity. This implies that the United States cannot decouple its oil and gas production and consumption from other countries. For example, a disruption in the Strait of Hormuz leading to the Persian Gulf will affect about 40 percent of the globe’s oil deliveries because of shipments from Iran, Iraq, Kuwait, Qatar, Saudi Arabia, and the United Arab Emirate. Such a disruption might occur in an armed conflict with Iran, which has been at loggerheads with the United States over its nuclear program. Moreover, while the United States has not been importing significant amounts of oil from the Middle East recently, U.S. allies Japan and South Korea rely heavily on oil from that region. Thus, a major principle for U.S. national security is to work cooperatively with these allies to develop a plan to move away from overreliance on oil and gas from this region and an even longer term plan to transition away from fossil fuels.
Actually, this long term plan is not really that far into the future. According to optimistic estimates (for example, from Cambridge Energy Research Associates) for when global oil production will reach its peak, the world only has until at least 2030 before the peak is reached, and then there will be a gradual decline in production over the next few decades after the peak.1 (Pessimistic views such as from oil expert Colin Campbell predict the peak occurring around 2012 to 2015.2 We thus may already be at the peak.) Once the global decline starts to take effect, price shocks could devastate the world’s economy. Moreover, as the world’s population is projected to increase from seven billion people today to about nine billion by mid-century, the demand for oil will also significantly increase given business as usual practices.
For the broader scope national security reason of having a stable economy, it is imperative to develop a nonpartisan plan for transitioning from the “addiction” to oil that President George W. Bush called attention to in his State of the Union Address in January 2006. While skepticism about the science of climate change will prevail, this should not hold back the United States working together with other nations to craft a comprehensive energy plan that saves money, creates more jobs, and overall strengthens international security.
FAS is developing a new project titled Sustainable Energy and International Security. FAS staff will be contacting experts in our network to form a diverse group with expertise in energy technologies, the social factors that affect energy use, military perspectives, economic assessments, and security alliances. I welcome readers’ advice and donations to start this project; please contact me at cferguson@fas.org. FAS relies on donors like you to help support our projects; I urge you to consider supporting this and other FAS projects.
Thinking More Clearly About Nuclear Weapons: The Ukrainian Crisis’ Overlooked Nuclear Risk
The destructive potential of nuclear weapons is so great that decisions impacting them should be made in a fully conscious, objective manner. Unfortunately, there is significant evidence that this is not the case. One of my Stanford course handouts1 lists almost two dozen assumptions which underlie our nuclear posture, but warrant critical re-examination. This column applies that same kind of analysis to the current Ukrainian crisis.
It is surprising and worrisome that almost none of the mainstream media’s coverage of the Ukrainian crisis has mentioned its nuclear risk. With the West feeling that Russia is solely to blame, and Russia having the mirror image perspective, neither side is likely to back down if one of their red lines is crossed. Add in America’s overwhelming conventional military superiority and Russia’s 8,000 nuclear weapons, and there is the potential for nuclear threats. And, where there is the potential for nuclear threats, there is also some potential for nuclear use.
I’m not saying a repeat of the Cuban Missile Crisis is likely, but given the potential consequences, even a small risk of the Ukrainian crisis escalating to nuclear threats would seem too high.
The frequency with which we find ourselves in such confrontations is also a factor. A low probability nuclear risk that occurs once per century is ten times less likely to explode in our faces than one that occurs once per decade. And the latter hypothesis (confrontations occurring approximately once per decade, instead of once per century) is supported by the empirical evidence, as the Georgian War occurred just six years ago.
While both Russia and the West are wrong that the current crisis is solely the other side’s fault, this article focuses on our mistakes since those are the ones we have the power to correct.
An example of the West’s belief that the crisis is all Russia’s fault appeared in a July 18 editorial 2 in The New York Times which claimed, “There is one man who can stop [the Ukrainian conflict] — President Vladimir Putin of Russia.”
Another example occurred on September 3, when President Obama stated: “It was not the government in Kyiv that destabilized eastern Ukraine; it’s been the pro-Russian separatists who are encouraged by Russia, financed by Russia, trained by Russia, supplied by Russia and armed by Russia. And the Russian forces that have now moved into Ukraine are not on a humanitarian or peacekeeping mission. They are Russian combat forces with Russian weapons in Russian tanks. Now, these are the facts. They are provable. They’re not subject to dispute.” (emphasis added)
So what’s the evidence that the New York Times and the president might be wrong? In early February, when the crisis was in its early and much less deadly stages, Ronald Reagan’s Ambassador to Moscow, Jack Matlock, wrote3: “I believe it has been a very big strategic mistake – by Russia, by the EU and most of all by the U.S. – to convert Ukrainian political and economic reform into an East-West struggle. … In both the short and long run only an approach that does not appear to threaten Russia is going to work.” (emphasis added)
A month later, on March 3, Dmitri Simes, a former adviser to President Nixon, seconded Ambassador Matlock’s perspective when he said in an interview4: “I think it [the Obama administration’s approach to the Ukraine] has contributed to the crisis. … there is no question in my mind that the United States has a responsibility to act. But what Obama is doing is exactly the opposite from what should be done in my view.”
Two days later, on March 5, President Nixon’s Secretary of State and National Security Adviser, Henry Kissinger, wrote:5 “Each [Russia, the West, and the various Ukrainian factions] has made the situation worse.”
A number of other articles by foreign policy experts also question the Times and President Obama placing all the blame for the crisis on Russia, but I hope I’ve made the point that Putin is not the only man who could end the fighting. Indeed, he may not be capable of doing that without us also correcting some of our mistakes.
Further evidence that the New York Times and President Obama might be wrong can be found in an intercepted and leaked phone conversation6 in which Estonia’s Foreign Minister, Urmas Paet – clearly no friend of Russia’s – stated that the sniper fire on February 20, which killed dozens of Maidan protesters and led to calls for Yanukovych’s head, appeared to have been a false flag operation perpetrated by the most violent elements within the protesters – for example, the ultra-nationalist Right Sektor, which is seen as neo-Nazi in some quarters.
Here is the exact wording of Paet’s key allegation in that phone call: “There is now stronger and stronger understanding that behind [the] snipers … it was not Yanukovych, but it was somebody from the new coalition.” That “new coalition” is now the Ukrainian government.
While this allegation has received little attention in the American mainstream media, German public television sent an investigative reporting team which reached the same conclusion7: “The Kiev Prosecutor General’s Office [of the interim government] is confident in their assessment [that Yanukovych’s people are to blame for the sniper fire, but] we are not.”
This is not to say that Paet and the German investigators are correct in their conclusions – just that it is dangerously sloppy thinking about nuclear matters not to take those allegations more seriously than we have.
While Putin has exaggerated the risk to ethnic Russians living in Ukraine for his own purposes, the West has overlooked those same risks. For example, on May 2 in Odessa, dozens of pro-Russian demonstrators were burned alive when an anti-Russian mob prevented them from fleeing the burning building into which they had been chased. According to the New York Times8: “The pro-Russians, outnumbered by the Ukrainians, fell back … [and] sought refuge in the trade union building. Yanus Milteynus, a 42-year-old construction worker and pro-Russian activist, said he watched from the roof as the pro-Ukrainian crowd threw firebombs into the building’s lower windows, while those inside feared being beaten to death by the crowd if they tried to flee. … As the building burned, Ukrainian activists sang the Ukrainian national anthem, witnesses on both sides said. They also hurled a new taunt: “Colorado” for the Colorado potato beetle, striped red and black like the pro-Russian ribbons. Those outside chanted “burn Colorado, burn,” witnesses said. Swastikalike symbols were spray painted on the building, along with graffiti reading “Galician SS,” though it was unclear when it had appeared, or who had painted it.”
Adding to the risk, on August 29, Ukraine took steps to move from non-aligned status to seeking NATO membership, and NATO’s Secretary-General Anders Fogh Rasmussen said he would “fully respect if the Ukrainian parliament decides to change that policy [of non-alignment].” Somewhat paradoxically, it is extremely dangerous – especially for Ukraine – for Rasmussen to encourage its hopes of joining NATO since Russia would likely respond aggressively to prevent that from occurring.
Also adding to the danger is an escalatory spiral that appears to be in process, with NATO taking actions that are seen as threatening by Russia and Russia responding in kind, with Putin reminding the world9 that, “Russia is one of the most powerful nuclear nations. This is a reality, not just words.”
We also need to question whether it is in our national security interests to ally ourselves with the Kiev government when, on September 1, its Defense Minister declared that, “A great war has arrived at our doorstep – the likes of which Europe has not seen since World War Two.”10
Also on September 1, a group of former CIA intelligence analysts warned11that: “Accusations of a major Russian invasion of Ukraine appear not to be supported by reliable intelligence. Rather, the intelligence seems to be of the same dubious, politically fixed kind used 12 years ago to justify the U.S.-led attack on Iraq.” (The group also warned about faulty intelligence in the lead-up to the Iraq War.)
These former intelligence analysts are not saying that our government’s accusation is wrong. But they are reminding us that there is historical evidence indicating that we should be more cautious in assuming that it is correct.
In a September 4 article in Foreign Policy, “Putin’s Nuclear Option,” 12 Jeffrey Taylor argues that: “Putin would never actually use nuclear weapons, would he? The scientist and longtime Putin critic Andrei Piontkovsky, a former executive director of the Strategic Studies Center in Moscow and a political commentator for the BBC World Service, believes he might. In August, Piontkovsky published a troubling account of what he believes Putin might do to win the current standoff with the West – and, in one blow, destroy NATO as an organization and finish off what’s left of America’s credibility as the world’s guardian of peace.”
I strongly encourage readers to read the full article. Again, Piotkovsky’s scenario is not likely, but given the consequences, even a small risk could be intolerable.
Defusing the Ukrainian crisis will require a more mature approach on the part of all parties. Focusing on what we need to do, we need to stop seeing Ukraine as a football game that will be won by the West or by Russia, and start being concerned with the safety of all its residents, of all ethnicities. If we do that, we will also reduce the risk that we find ourselves repeating the mistakes of the Cuban Missile Crisis, when neither side wanted to stare into the nuclear abyss, but both found themselves doing so.
As noted earlier, our mishandling of the Ukrainian crisis is unfortunately just one instance of a larger problem – dangerously sloppy thinking about nuclear weapons. Given that the survival of our homeland is at stake, our government needs to undertake a top-to-bottom review of the assumptions which underlie our current nuclear posture and correct any that are found to be wanting.
Dr. Martin E. Hellman is an Adjunct Senior Fellow for Nuclear Risk Analysis at FAS. Hellman was at IBM’s Watson Research Center from 1968-69 and an Assistant Professor of EE at MIT from 1969-71. Returning to Stanford in 1971, he served on the regular faculty until becoming Professor Emeritus in 1996. He has authored over seventy technical papers, ten U.S. patents and a number of foreign equivalents.
Along with Diffie and Merkle, Hellman invented public key cryptography, the technology which allows secure transactions on the Internet, including literally trillions of dollars of financial transactions daily. He has also been a long-time contributor to the computer privacy debate, starting with the issue of DES key size in 1975 and culminating with service (1994-96) on the National Research Council’s Committee to Study National Cryptographic Policy, whose main recommendations have since been implemented.
His current project, Defusing the Nuclear Threat, is applying quantitative risk analysis to a potential failure of nuclear deterrence. In addition to illuminating the level of risk inherent in threatening to destroy civilization in an effort to maintain the peace, this approach highlights how small changes, early in the accident chain, can reduce the risk far more than might first appear. This methodology has been endorsed by a number of prominent individuals including a former Director of the National Security Agency, Stanford’s President Emeritus, and two Nobel Laureates.
A Looming Crisis of Confidence in Japan’s Nuclear Intentions
Nearly two years into Prime Minister Shinzo Abe’s second stint at governing Japan, his tenure has been characterized by three primary themes. The first two themes include his major legislative priorities: enabling Japan’s economic revival and bringing Japan closer to the status of a “normal” country that takes on a greater share of its own security needs. Both of these priorities are largely celebrated in the United States, which longs to see Japan become a more able and active partner in the region. A third theme has not been well received in Washington: the prime minister’s apparent efforts to whitewash Japan’s wartime past. Through personal expressions of admiration for convicted war-criminals, an official reinvestigation of past apologies for war-time atrocities, and appointments of hardline nationalists to prominent posts (such as the NHK board of governors), the prime minister’s actions have raised the spectre among wary neighbors of a Japanese return to militarism and begun raising eyebrows even among friends in Washington.
It is against this backdrop that Japan is now attempting to reinstate its nuclear energy program. Japan, which, not long ago, had planned to generate half of its electricity from nuclear power by 2030, has watched its nuclear reactors sit largely idle since the Fukushima disaster in 2011. Abe’s government views nuclear restarts as a critical pillar of his first legislative priority—Japan’s economic recovery. However, observers both outside and inside Japan note that, in addition to providing Japan the baseload electricity that its economy craves, the country’s sophisticated nuclear energy program effectively serves a dual purpose, providing Japan a latent nuclear weapons capability as well.
Citing Abe’s particular treatment of historical issues, some have begun to question whether reinstatement of Japan’s nuclear program is really more about the prime minister’s security goals than his economic agenda. China, for one, has hinted at allegations of a Japanese nuclear weapons program—after a recent incident in which Japan negotiated to repatriate an aging store of highly enriched uranium (HEU) to the United States, Chinese media propagated a narrative that twisted the event into evidence of Japan’s militaristic intentions.1 Koreans have begun expressing similar concerns.2
In fact, Japan’s current movement towards a more normal military posture is not entirely unrelated to the push to restart the country’s nuclear energy program—it was the Fukushima nuclear disaster that both idled Japan’s nuclear fleet and helped enable the return of the more hawkish LDP government. But the relationship likely ends there. As a legacy of World War II, Japanese society’s discomfort with the idea of a “normal” Japan has restricted Abe’s normalization efforts to steps that are only modest by any comparable measure.3 Events that have conspired to suggest the possibility of Japanese nuclear weapons are reflective of awkward timing and, perhaps, less than acute politics, but not likely of some new militant spirit in Japanese society. Unfortunately, as Japan pushes to restart its nuclear energy program in the months and years ahead, circumstances are aligning that will amplify—not mitigate—alarm over Japan’s nuclear intentions.
Japan’s Plutonium Economy
For a tangle of social and legal reasons, the restart of Japanese reactors is tied together with operation of Japan’s nuclear fuel reprocessing plant at Rokkasho Village in Aomori Prefecture. Under agreements with reactor host communities, utilities cannot operate reactors unless there is somewhere for nuclear fuel to go once it has been used. Because Japan lacks a geological repository and nearly all plant sites lack dry cask storage facilities,4 Rokkasho is currently the only viable destination for spent fuel from Japan’s reactors. Unless this situation changes, Japan is effectively unable to operate reactors without Rokkasho.
Rokkasho itself is, in turn, effectively dependent on Japan’s operating reactors. According to a sort of public-private arrangement that has been in place since before Fukushima, Japanese utilities send spent nuclear fuel to Rokkasho, where it is separated into waste and fissionable MOX (mixed uranium and plutonium oxides) powder. MOX is processed into fresh reactor fuel and sent back to Japan’s reactors. High-level waste is ultimately sent to a geological repository that is to be built in a different prefecture (one of Aomori Prefecture’s conditions for originally agreeing to host the reprocessing plant). Of Japan’s reactors, 16 to 18 of the 54 that were operating prior to the Fukushima accident would, after receiving local government consent, consume MOX in an effort to maximize use of Japan’s limited energy resources. That was the plan—prior to the Fukushima disaster, anyway.
As a legacy of the Fukushima disaster, Japan’s nuclear reactors currently sit idle. The six at Fukushima Daiichi will never operate again, nor will a number of others that are older, particularly vulnerable to earthquakes and tsunamis, or for other reasons not worth the trouble and expense of restarting. While impossible to predict for certain, a consensus seems to be emerging among experts and industry watchers that post-Fukushima, somewhere in the order of half of Japan’s original 54 reactors will return to service under Japan’s new regulatory regime. Currently, two reactors (Sendai 1 and 2 in southwestern Japan) have cleared safety reviews from Japan’s new regulator, the Japan Nuclear Regulatory Authority (JNRA), and now appear headed towards restart this winter. Eighteen more reactors await review from the JNRA. Of those 20 reactors, only five5 have received consent to use MOX, but that was prior to Fukushima. All 20 reactor restarts depend on the promise of a functioning Rokkasho. But if Rokkasho were to restart on a similar timeframe as the reactors, one thing is certain—there will be far fewer than the originally envisioned 16 to 18 reactors available to consume the MOX when the plant starts up.6
Reactor Restart X-Factors
As with Rokkasho, the question of when the JNRA will conclude its reviews of the next eighteen reactors remains quite murky. However, it stands to reason that ultimately most, if not all, of the reactors that have applied for restart will ultimately pass safety inspections. Japan’s electric power companies are unlikely to have invested the time and resources in plant upgrades and regulatory application had they less than a high degree of confidence that they would qualify under Japan’s new regime. Likewise, there is little question that Japan’s LDP government (assuming an LDP government at the time of restart) would stand in the way of restarts. But the JNRA and national government are only two of the three main factors in restarting Japan’s reactors—leaders of the towns and prefectures that host nuclear power plants have a de facto say in the matter as well.
The conventional wisdom is that local leaders have strong financial incentives to restart the nuclear power plants that they host: government and industry have historically lavished incentives on host communities and prefectures in order to overcome any inclination toward local resistance. In one sense, local governments have over time become dependent on plants and can ill afford to forego not only the government and utility incentives, but also the base of jobs and tax revenues they represent. On the other hand, communities need now only look to the example of the towns that have been rendered uninhabitable by the Fukushima disaster to see a terrifyingly clear picture of their tradeoff.
In some cases, apparently including the Sendai reactors, it is unlikely that local government would stand in the way of restarts. Earthquakes are less common in Kyushu,7 the geography on the west coast is less prone to large tsunamis, and local residents may take comfort in the fact that Sendai reactors are pressurized water reactors—not the boiling water rector type used at Fukushima Daiichi. But in other cases, local approvals may not be as certain. Take for example TEPCO’s Kashiwazaki-Kariwa plant in Nagano prefecture, where Governor Izumida has very publicly challenged TEPCO. He has insisted that, irrespective of the findings of the JNRA, with the Fukushima Daiichi reactor cores still too highly radioactive to investigate and verify the true nature of the accident, he will be unwilling to allow the Kashiwazaki-Kariwa reactors to restart.
In addition to the local government factor, an X-factor may be emerging—preemptive lawsuits against reactor restarts. Earlier this year, in Fukui prefecture where political leadership otherwise favors nuclear power, a citizens group brought a lawsuit alleging an inadequate basis for confidence in the restart of the Oi plant.8 More recently, a second lawsuit has been brought by the city of Hakodate (Hokkaido prefecture) against the yet-to-be completed Ohma plant in nearby Aomori prefecture.9 In the case of Oi, a local judge sided with the plaintiffs, but the decision has been appealed by Kansai Electric Power Company, and the case is all but certain to drag out until long past the serviceable lifetime of the Oi reactors. The Hakodate case is ongoing.
It is possible that Governor Izumida is an outlier and that the Fukui and Hakodate challenges will prove to be ineffective and isolated. However, it is equally possible that there are more Governor Izumidas and lawsuits yet to come. Furthermore, what is undeniable is that these cases have set a precedent and raised public pressure on local officials to seriously consider opposing restart of local reactors even if they do pass JNRA safety inspections. In any case, it is premature to presume that once the JNRA has rendered a safety verdict, reactor restart is imminent.
The MOX Question
Within the concurrent push to open Rokkasho and restart reactors, the availability of MOX-burning reactors seems to be assumed. But, notwithstanding all of the other hurdles facing nuclear reactor restarts in Japan, MOX fuel itself has been a subject of controversy and public discomfort since even before the Fukushima disaster. As utilities received approvals to burn MOX fuel and subsequently began receiving shipments of MOX from Europe (where it had been processed on behalf of Japan’s utilities), they were met with consistent and passionate public protests. These protests were typically confined to a cohort of smaller national-level interest groups that argue that using MOX elevates risk in transportation and regular reactor operation.10 On a national scale, prior to Fukushima the fuel cycle has been a relatively fringe issue—MOX had been a largely unfamiliar acronym to the public. Post-Fukushima, as utilities push for restarts amidst an atmosphere of heightened public scrutiny, there will be no free pass for MOX. For nuclear energy opponents, the prospect of MOX usage would provide one more narrative with which to hammer against proposed reactor restarts.
At the macro level, utilities share in the incentive to burn MOX fuel as they depend on Rokkasho, and Rokkasho is hard to rationalize in the absence of a functioning MOX program. However, in a much more tangible and immediate sense, utilities desperately need their reactors up and running again. Most of Japan’s utilities have posted consistent losses since their reactors were relegated to nonperforming assets on their balance sheets and they were forced to substitute expensive fossil fuels for relatively cheaper nuclear power. For Japan’s utilities, restarting nuclear reactors could be a life or death proposition. That being the case, can it be taken for granted that utilities will risk complicating their restart efforts by forging ahead with plans to burn MOX? Will the government create explicit incentive for utilities to do so? Given enhanced public scrutiny, it cannot be assumed that the pre-Fukushima local approvals for MOX usage will be honored anyway.
The Japanese government’s 2014 energy policy (despite reaffirming Japan’s commitment to its beleaguered ‘no surplus plutonium’ policy), gives blessing to proceeding with Rokkasho (recognizing that, among other things, if it didn’t, Aomori threatens to send the spent nuclear fuel right back to the plants of origin). But even assuming that the five MOX reactors under regulatory review do receive restart approval and recommence MOX burning, the original goal of 16 to 18 Japanese reactors burning MOX fuel seems far off.11 There has been some suggestion that Rokkasho could restart slowly, at a throughput commensurate with the ability to consume MOX. However, as Meiji University Professor Tadahiro Katsuta points out, reducing throughput of Rokkasho effectively raises the per-unit cost of MOX, necessitating a reexamination of the cost basis on which the MOX program was justified to Japanese ratepayers.12
Rokkasho Controversy
Even outside of the MOX capacity question, Rokkasho is not without controversy. Officially, Rokkasho is justified as an investment in energy security for Japan. However, from the standpoint of global nonproliferation concerns, Japan sets an uncomfortable precedent with Rokkasho. While otherwise a leading global champion for peace and nuclear disarmament, Japan is the only non-nuclear weapons country to possess a commercial nuclear fuel recycling program. Whereas global nonproliferation efforts prioritize limiting the spread of reprocessing capabilities, Rokkasho has enabled Iran, for one, to point to Japan in defending the legitimacy of its own fuel cycle activities. South Korea, seeking American consent for a Korean recycling program, also cites Japan’s example in negotiating a replacement for the U.S.-ROK nuclear cooperation agreement that expires in 2016.
Controversial or not, Japan’s leaders feel compelled to push forward with Rokkasho and through an agreement under section 123 of the Atomic Energy Act,13 they enjoy the support of the United States government. American consent to Rokkasho is only guaranteed through 2018, but the United States, which granted consent in 1988 largely out of deference to diplomatic concerns, for the same reason is highly unlikely to withdraw consent in 2018. Given the effective concurrence of the 2018 date with U.S.-ROK negotiations and the looming startup of Rokkasho in the face of low (or no) capacity to consume MOX, timing has become extremely awkward.
As Rokkasho proceeds towards restart, public reaction from Washington has been surprisingly muted. Perhaps this reflects appreciation for the energy conundrum in which Japan finds itself, or tacit consent that bringing Japan’s nuclear industry back onto solid footing after the Fukushima disaster was always going to be awkward—Japan has an inherent chicken or egg dilemma in restarting Rokkasho and its reactors. But the reality is that Japan’s situation puts Washington in a very tough spot. Washington is effectively complicit in what might appear to be Japanese disregard for its own commitments to global nonproliferation. This poses a risk to the global nonproliferation regime and American credibility on the subject.
Implications
Global nonproliferation principles undoubtedly remain a high priority for Japan. But it is likely that in the short term, the eyes of Japan’s leaders are focused more intently on bringing nuclear reactors back on line. Particularly in the context of Prime Minister Abe’s provocative views on history, the perception outside of Japan is certain to be one of alarm if Japan is seen to be separating plutonium without a credible pathway for its disposition. While the coincidence of the 2016/2018 Korea and Japan 123 agreements and Japan’s reentry into nuclear energy will shine a spotlight on the American role in Japan’s nuclear fuel cycle scheme, it is seen as highly unlikely that the United States will attempt to withdraw from or renegotiate the 123 agreement with Japan irrespective of Japan’s plutonium balance concerns. This will effectively make the United States appear complicit in Japan’s growing inventory of plutonium.
For the United States, this situation has consequences on three fronts. Firstly, Japan’s apparent failure to abide by its plutonium commitments undercuts American interests in limiting fuel cycle capabilities through treaty agreements. Nowhere is this more obvious than in the ongoing U.S.-ROK 123 agreement negotiations. Secondly, Japan is a leader, if not the symbolic face of the global nonproliferation regime. For Japan to be separating plutonium for no demonstrable purpose dramatically undercuts its own leadership on nonproliferation and aggravates the already controversial precedent it sets with its fuel cycle program, elevating the risk of proliferation in the region. Thirdly, at just the time when the United States is working to underscore its alliance with Japan as the bedrock of its security presence in East Asia, Japan’s growing plutonium surplus will only exacerbate concerns of Japan’s return to militarism, eroding its legitimacy and efficacy as a partner in regional security.
In the aftermath of the Fukushima disaster, the United States has appeared somewhat ambivalent in its response to Japan’s efforts to restart its nuclear energy system. However, the American stake in Japan’s road ahead is profound. While it is not the case in all foreign capitals, in Tokyo, opinions and preferences from Washington are meaningful. Washington, particularly the Department of State and Department of Energy, has an opportunity to protect American interests by formulating and articulating an unambiguous American position on Japan’s path forward on nuclear energy to Japan’s leadership.
The critical interest of the United States would be for Japan to demonstrate clear commitment to the no-surplus plutonium policy and to the global nonproliferation regime. As elements of a policy that might be necessary to make that happen, the United States should urge Japan’s leadership and utilities to:
- Articulate a plan for plutonium disposition that provides quantifiable and publicly demonstrable benchmarks for reducing Japan’s plutonium inventory.
- Call for official, temporary suspension of operations at Rokkasho until MOX burners or another credible disposition pathway for Japan’s separated fissile materials, is available.
- Advocate operating Rokkasho (if and when started), at an output rate that is no more than commensurate with plutonium disposition goals and available means for plutonium disposition.
- Encourage utilization of temporary dry-cask storage of spent nuclear fuel in order to enhance safety at reactor sites while expanding nuclear fuel cycle policy options. One of the rare positive stories to emerge from the Fukushima Daiichi disaster was the robustness of dry cask storage. Utilities and the government should capitalize on this success story and prioritize arrangements with local communities to allow for expeditious transfer of spent nuclear fuel from wet-storage to on-site dry casks.
There is no nuclear weapons program in Japan’s foreseeable future. However, there is a significant risk of an outward appearance that suggests otherwise to South Korea, China, North Korea, Iran, and the rest of the world. Whether or not appearance differs from reality, the real world consequences would likely be the same. While Japan has serious and immediate energy concerns, it also has a very deep and fundamental commitment to global nonproliferation. With support from friends in Washington, Japan must face its looming nuclear energy challenges head on with eyes fully open. The stakes are too high to allow current circumstances to dictate their own outcomes.
Next year is the 70th anniversary of the atomic bombings of Hiroshima and Nagasaki. The event would provide a fitting platform for Prime Minister Abe to recognize opportunities in Japan’s current crisis and make bold decisions on Japan’s nuclear energy program. The right decisions can help regain global confidence in Japan’s intentions, while reminding the world of Japan’s unwavering commitment to nuclear safety and nonproliferation. The anniversary would make an equally unfortunate occasion to demonstrate otherwise.
Ryan Shaffer is an Associate Director of Programs at the Maureen and Mike Mansfield Foundation in Washington, D.C., where he manages Japan and Northeast Asia policy programs including the Mansfield-FAS U.S.-Japan Nuclear Working Group. Prior to joining the Mansfield Foundation, Mr. Shaffer served as a research analyst for the Federation of Electric Power Companies of Japan.
The CTBT: At the Intersection of Science and Politics
Recent high-level meetings in Washington, D.C., the United Nations, California and Utah about the Comprehensive Test Ban Treaty (CTBT) might lead one to believe that finally action might be taken towards ratification of the treaty. At the meeting in New York, foreign ministers and senior officials from 90 countries met on September 29 to acclaim the treaty and its value—both scientific and political. “This treaty isn’t just a feel-good exercise. It’s in all of our national security interests, and it’s verifiable,” said U.S. Secretary of State John Kerry. “In fact, its verification regime is one of the great accomplishments of the modern world.” 1
In Washington, key U.S. officials—Secretary of Energy Ernest Moniz, NNSA Director Frank Klotz, Under Secretary of State for Arms Control and International Security Rose Gottemoeller, Assistant Secretary of Defense for Nuclear, Chemical and Biological Programs Andrew Weber–as well as Executive Secretary of the CTBT Organization Preparatory Commission Lassina Zerbo, held a public meeting on nuclear testing on September 15. “Today we can say with even greater certainty that we can meet the challenges of maintaining our stockpile with continued scientific leadership, not nuclear testing,” said Secretary Moniz. “So again, I repeat that the United States remains committed to ratification and entry into force of the Comprehensive Nuclear-Test-Ban Treaty, along with the monitoring and verification regime. And this administration will continue making the case for U.S. CTBT ratification to build bipartisan support.”2
Referring to the United States supercomputing and other capabilities, Mr. Klotz noted, “Thanks to this effort, today we have a greater understanding of how nuclear weapons actually work than we did when we were carrying out nuclear explosive testing. This is a remarkable achievement in innovation for our national security, and it is foundational to an effective no-test regime.”3
In addition, the largest scale, on-site inspection (OSI) simulation to date will be launched for a month in November in Jordan, and includes a day of visiting dignitaries, possibly to include the king. An OSI is the final step in the verification regime; it is a complex endeavor that involves enormous amounts of equipment and scientific as well as operational expertise. The simulation will be a significant test of the system.
Politics meets Science
Although the treaty deals with the highly technical and sensitive subject of nuclear test explosions, it has been considered in a political context since the negotiations. Countries possessing nuclear weapons do not want others to know about their facilities or capabilities, so verification provisions of the treaty were exceptionally difficult to negotiate. World-renown scientists worked in their labs and academic institutions, as well as in Geneva, on the intricacies of devising an international monitoring regime. They advised some of the top diplomats negotiating the treaty in Geneva. Even now, those working on the treaty still grapple with scientific questions in a political setting, the CTBTO Preparatory Commission in Vienna, as well as in their labs or government offices. The scientists possess the expertise and deep knowledge of the various verification techniques, and they provided information and counsel to their ambassadors, but the diplomats make the decisions.
Originally the key issues in the treaty revolved around questions such as: who should be included in the negotiations? What was to be the scope of the ban—a few pounds equivalent TNT of explosive yield, hundreds, or zero? (Negotiators decided on zero.) Would the non-nuclear weapon states agree with the nuclear weapon states that this would be a treaty to “ban the bang, not the bomb?” What kinds of monitoring technologies should be included in the treaty verification regime? Where would monitoring stations be placed? How many stations would be installed in the nuclear weapon states? Who would decide to conduct an OSI, and how would it be conducted? How could access to sensitive facilities be managed? And a crucial question: which countries were essential for entry into force? All of these questions, and more, involve complex scientific issues, but are highly political and contentious.
The CTBT prohibits “any nuclear weapon test explosion or any other nuclear explosion” anywhere in the world. The verification provisions of the CTBT are more extensive and intrusive than those of other treaties, as they are designed to detect nuclear explosions anywhere on the planet: underground, in the oceans, and in the atmosphere. In order to verify compliance with its provisions, the treaty specifies a global network of 327 monitoring facilities in strategic locations in 89 countries, and allows for on-site inspections of suspicious events. The International Monitoring System (IMS) comprises four complementary or synergistic technologies: seismic, radionuclide, hydroacoustic and infrasound. The 50 primary and 120 auxiliary seismic stations monitor the ground for shockwaves that are caused by nuclear explosions. A radionuclide network encompassing 80 stations uses air samplers to detect radioactive particles released from atmospheric, underground or underwater explosions. Half of these stations will be capable of detecting noble gases upon entry into force of the treaty. In addition, 16 radionuclide laboratories will analyze samples of filters from the stations (evidence of radionuclides is called the “smoking gun” of a nuclear explosion). In addition, there are 11 hydroacoustic stations to detect explosions in the oceans, and 60 infrasound stations designed to detect nuclear explosions in the atmosphere.
The CTBT Organization (CTBTO) Preparatory Commission was established in March 1997 and has been building up the verification regime, which is about 90 percent complete. The IMS has been detecting global activities such as: some 100 earthquakes each day, the nuclear test explosions conducted by North Korea in 2006, 2009, and 2013, in addition to tsunamis, the dispersal of the radioactive plume from Fukushima, and other activities. Data from the IMS stations is transmitted for analysis to the International Data Center of the Preparatory Commission’s Provisional Technical Secretariat.
In November 2014, the Preparatory Commission will embark on its second full-scale on-site inspection (OSI) simulation in Jordan to test the operation and techniques of an OSI in an integrated manner. Although the treaty includes a timeline and specifics of the on-site inspection regime, details of the operational manual and other logistical arrangements were left for the Preparatory Commission to elaborate. The exercise in Jordan will be the most challenging endeavor to date, aimed to test the ability to conduct an on-site inspection under realistic and challenging conditions, and to demonstrate the progress made since the last Integrated Field Exercise in Kazakhstan in 2008 (IFE08). During Integrated Field Exercise 2014 (IFE14), the Preparatory Commission will simulate a CTBT on-site inspection in which 40 inspectors search a designated area of up to 1,000 square kilometers for evidence of a possible nuclear explosion. IFE14 aims to test 15 of the 17 inspection techniques listed in the treaty in an integrated manner, a significant increase in the technologies tested at the previous IFE in Kazakhstan.
There are numerous OSI techniques, from visual observations and over-flights to multi-spectral imaging, airborne gamma spectroscopy, gamma radiation monitoring, environmental sampling, measurement of argon-37 and radioxenon, seismological monitoring of aftershocks, magnetic field mapping, resonance seismometry, and drilling, among others. The IFE14 will not employ drilling (which is extremely expensive), or resonance seismometry. It plans to exercise each phase of an OSI, from the receipt of the request through the launch, pre-inspection and post-inspection activities, reporting preliminary findings, departure, and return of equipment and personnel. The exercise will involve transporting some 150 tons of equipment from Austria to Jordan. According to the PTS website, the IFE14 will also test newly developed operational elements of an OSI such as an enhanced operations support center, an improved in-field communications system and a rapid deployment system for transporting tons of inspection equipment anywhere in the world.4It should be noted that an OSI can only take place once the treaty has entered into force, thus the Preparatory Commission has had time to develop the procedures.
The provisions in the treaty for an OSI are quite demanding. Because some of the effects from a nuclear explosion can be short-lived, the Executive Council will need to decide on the on-site inspection request within 96 hours of its receipt from the requesting state party, and an OSI inspection team and its equipment must be deployed and initiate inspection activities within a six-day period. During the course of the inspection, the inspection team may submit a proposal to extend the inspection to begin drilling, which must be approved by 26 Council members. The inspection may continue for 60 days, but if the inspection team determines that more time is needed to fulfill its mandate, it may be extended by an additional maximum of 70 days, subject to Council approval.
Developments since 1996
Under the terms of the treaty, countries can use data outside of the IMS that is available from thousands of other seismological or radionuclide stations, satellite observations and other national technical means, as well as advanced data analysis in their efforts to monitor the treaty. Countries may submit this additional information as a basis for a request for an OSI. The IMS is performing far better than expected by the negotiators who designed it. Yet since the CTBT was negotiated in 1996, dramatic scientific and technical advances in monitoring and data analysis techniques have occurred. If countries use the data from the IMS, in combination with what is available outside of the IMS, they could enhance their monitoring capability significantly beyond that provided by the treaty, by an order of magnitude. Using this combination of information would also allow a country to focus its monitoring efforts on countries of concern, individually or in cooperation with others. Such “precision monitoring” would be useful to countries of a region that could pool their resources to support the effort.5 In contrast, the Technical Secretariat is not allowed to use information from stations or advanced technologies that are not included in the treaty. Further, the Secretariat must take a global approach; it is not allowed to focus on specific areas. Such precision monitoring capabilities would be of interest to states where verification might be an issue in the ratification process.
By focusing on specific areas of concern, precision monitoring would also increase the ability to accurately locate events. This is key to identifying areas for on-site inspections. A notable feature of the CTBT is that the decision regarding non-compliance or whether to conduct an on-site inspection rests with the States Parties, not the CTBTO Technical Secretariat. This is contrary to the role of the IAEA and the Nuclear Nonproliferation Treaty (NPT). It is therefore in the interest of the international community to expand the knowledge base on verification technologies in all countries in order to improve their ability to monitor the Treaty. Countries will need to send to Vienna representatives who are well versed in the provisions of the treaty and who have scientific expertise in the technologies it employs. This will increase the likelihood that a vote in the Executive Council (responsible for compliance with the treaty) on an on-site inspection or a decision about non-compliance will be based on scientific facts rather than concentrating on political concerns. That is especially important, given that the decision to conduct an OSI must be made by 30 of the 51 members of the Executive Council. Critics of the treaty believe that it will likely be impossible to achieve such a number, although the criteria for membership in the Council are such that the nuclear weapon states will always have a seat on the Council. Although the United States and a few other countries have their own technical means to monitor compliance with the treaty, most countries have not yet established their national facilities to efficiently monitor it.
The treaty was opened for signature in September 1996, and has been signed by 183 nations and ratified by 163. The treaty cannot enter into force until it is ratified by 44 “nuclear capable” nations6 specified in the treaty, eight of which have yet to do so (China, Egypt, India, Iran, Israel, North Korea, Pakistan, and the United States). India, Pakistan, and North Korea have not signed it. Although many countries in the negotiations wanted to have a numerical provision (e.g., 65 ratifications), the five nuclear-weapon states in particular insisted that each of the five–and other states capable of developing a bomb–be on board for the treaty to enter into force.
After the United States triggered the negotiations on a CTBT in 1993 and committed considerable political will to conclude it in 1996 at the multilateral Conference on Disarmament, the Senate refused to ratify the treaty in 1999. Since then it has been sitting on the back burner of the U.S. Senate and its counterparts in the seven other countries required for entry into force. It is unlikely that the Obama administration will bring it up for a vote in the Senate unless it knows it has the votes. Only 28 of the senators who voted on the ratification of the treaty in 1999 remain in the Senate (13 of whom voted against), and that figure may decrease in the November elections. A number of the other holdout countries claim to be waiting for the United States to ratify first, which makes entry into force a political exercise waiting to happen. As Dr. Zerbo said in Washington, “We have to be mindful of those who have signed and ratified this treaty long ago, and been waiting for its entry into force.”
China is the only other nuclear-weapon state among the five declared under the NPT that still needs to ratify. “The U.S. pushed for the negotiations and it has tested more than any other nation,” said Sha Zukang, former Ambassador to the negotiations. “I firmly believe that, were the U.S. to ratify the Treaty, China would definitely follow.” He added that the CTBT could be useful in promoting China-U.S. strategic mutual trust and building a “new model of major country relations.” Noting that the United States had conducted 1032 nuclear test explosions and China and the UK just 45 each, he claimed that China and the international community believed that the United States “wanted to ensure the overwhelming superiority of its nuclear arsenal, both in quantity and quality.” He added, “Serious efforts should be made to encourage U.S. law-makers to change the idea of seeking absolute security at the cost of leaving all other countries feeling insecure, and then to support Treaty ratification.”7
In an influential 2009 report on the Strategic Posture of the United States8, conducted by a bipartisan Congressional Commission and chaired by former Secretary of State James Schlesinger, and former Secretary of Defense William Perry, the CTBT was the only issue on which agreement could not be achieved. Opponents contended that the zero yield prohibition of the treaty cannot be verified and that other countries would cheat, thereby gaining a military advantage, while the United States observed the terms of the treaty. They faulted the fact that the treaty does not define a nuclear test, and this could result in different countries having different understandings of prohibitions and restrictions, to include the possibility of conducting tests with hundreds of tons of nuclear yield. In this context, they believe that Russia and possibly China are conducting low yield testing. They also believe that maintaining a safe, reliable stockpile of nuclear weapons will require testing over time.9 A number of senators and decision makers believe these and other aspects of the treaty will not confer benefits and would pose security risks to the United States.10 Proponents contend that the treaty is a strong nonproliferation tool which is verifiable, and that other states could develop and test new or improved weapons without constraints (posing a greater threat to U.S. security), and that the United States can maintain a safe, secure, and reliable nuclear weapons stockpile without additional testing.
A number of studies have been conducted on the scientific and verification capabilities of the treaty, including one by the U.S. National Academy of Sciences. The Academy released in March 2012 an update of its 2002 report on Technical Issues associated with the Comprehensive Test Ban Treaty. The newer study, conducted by eminent scientists in the field, found that the United States “has the technical capabilities to maintain a safe, secure, and reliable stockpile of nuclear weapons into the foreseeable future without nuclear-explosion testing” and “is now better able to maintain a safe and effective nuclear stockpile and to monitor clandestine nuclear-explosion testing than at any time in the past.” The study found that globally, the IMS seismic network provides complete coverage at magnitude 3.8 with about 80 percent of stations operational.11 This is a low magnitude, and the capability has likely improved since the study was conducted, with 90 percent of the stations now operational. As mentioned previously, the IMS detected all three low yield tests conducted by North Korea. “Other States intent on acquiring and deploying modern, two-stage thermonuclear weapons would not be able to have confidence in their performance without multi-kiloton testing,” the report states. “Such tests would likely be detectable (even with evasion measures) by appropriately resourced U.S. national technical means and a completed IMS network.”
Scientific arguments notwithstanding, politics usually get in the way. As former NNSA Administrator Linton Brooks is fond of saying, “The CTBT will not be ratified until there is a Republican president who supports it.” Ambassador Brooks, who was a member of the more recent NAS committee, said that although the report presented positive conclusions about the capabilities of the treaty and was favorably received by both supporters and opponents for its technical accuracy, “I don’t know anyone whose mind it changed.”12
Following the negotiations, member states of the Preparatory Commission in Vienna established a Working Group on Verification to implement the verification regime. They began by considering issues such as what stations to build first, when data from the stations should be transmitted to the International Data Center in Vienna, how to configure the global communications infrastructure, how to authenticate the data, the elaboration of the OSI regime (techniques, equipment, training, operational manual), what the budget should be prior to entry into force, etc. The Working Group breaks down into sub-groups that are quite technical, if not wonkish. Scientific experts from many countries gather to consider topics such as sources of noble gas backgrounds, testing regional seismic travel time, data fusion, autonomous buoys, new optical seismometers, infrasound calibration, and the Bayesian inference approach. Again, the scientists who work in the technical sub-groups advise the diplomats who make decisions in the Working Group on these issues. The CTBTO Provisional Technical Secretariat then carries out these decisions. (In this context, it should be noted that three women participated in the scientific meetings in Geneva, two of whom are the only women participating in Vienna.)
Arguments over the treaty’s benefits and drawbacks have preceded the completion of the current treaty and go back to several earlier attempts decades ago at negotiating a ban. In fact, the negotiators of the Limited Test Ban of 1963 (LTBT) couldn’t agree on the verification provisions of a comprehensive ban, which is why it was limited (i.e. it covered all environments except underground). Article 1 of the CTBT is the same as Article 1 of the LTBT, but expanded to include underground. The LTBT has not been contested over the years, regardless of the fact that it has no verification provisions. Politics have trumped science in a number of instances, and the CTBT is no exception. Secretary Kerry said at the UN in September, “I know some members of the United States Senate still have concerns about this treaty. I believe they can be addressed by science, by facts, through computers and the technology we have today coupled with a legitimate stockpile stewardship program.” It remains to be seen if he can trump politics.
Jenifer Mackby is a Senior Fellow for International Security at FAS. Ms. Mackby has worked on international security, nonproliferation, and arms control issues at the Center for Strategic and International Studies (CSIS), the Comprehensive Nuclear-Test-Ban Treaty (CTBT) Organization, the Conference on Disarmament (CD) and the United Nations. As a Fellow and Adjunct Fellow at CSIS, she has led projects on U.S.-U.K. Nuclear Cooperation, Asian Trilateral Nuclear Dialogues, 21st Century Nuclear Issues, and the CTBT, and has worked on Strengthening the Global Partnership, European Trilateral Nuclear Dialogues, Reduction of U.S. forces in Europe, among others. Previously, she was responsible for the negotiations on the CTBT in the CD, as well as the Group of Scientific Experts that designed the core monitoring system of the treaty. She then served as Secretary of the Working Group on Verification at the CTBTO in Vienna. Ms. Mackby served as Secretary of the Biological Weapons Convention Review Conference, the UN Special Commission on Iraq, the Convention on Environmental Modification Techniques Review Conference, Nuclear Nonproliferation Treaty Review Conference committee, and others. She is a Senior Adviser for the Partnership for a Secure America, and has written extensively about non-proliferation and arms control issues.
Seismic Risk Management Solution for Nuclear Power Plants
Abstract
Nuclear power plants should safely operate during normal operations and maintain core-cooling capabilities during off-normal events, including external hazards (such as flooding and earthquakes). Management of external hazards to expectable levels of risk is critical to maintaining nuclear facility and nuclear power plant safety. Seismic risk is determined by convolving the seismic hazard with seismic fragilities (capacity of systems, structures, and components). Seismic isolation (SI) is one protective measure showing promise to minimize seismic risk.
Current SI designs (used in commercial industry) reduce horizontal earthquake loads and protect critical infrastructure from the potentially destructive effects of large earthquakes. The benefit of SI application in the nuclear industry is being recognized and SI systems have been proposed in American Society of Civil Engineer Standard 4, ASCE-4, to be released in the winter of 2014, for light water reactors facilities using commercially available technology. The intent of ASCE-4 is to provide criteria for seismic analysis of safety related nuclear structures such that the responses to design basis seismic events, computed in accordance with this standard, will have a small likelihood of being exceeded.
The U.S. nuclear industry has not implemented SI to date; a seismic isolation gap analysis meeting was convened on August 19, 2014, to determine progress on implementing SI in the U.S. nuclear industry. The meeting focused on the systems and components that could benefit from SI. This article highlights the gaps identified at this meeting.
Introduction
External hazards pose risks to nuclear power plants (NPPs) and nuclear facilities. Quantifying and managing this risk is important for safe operation of these facilities. Risk evaluations should follow a process similar to that shown in Figure 1; the process would start with risk-informed external hazard scenarios such as seismic, flood, fire, or tsunami, or a combination of these as initiating events. Verified and Validated (V&V) models would be used to simulate the external hazard initiators and model results would be used to determine which systems are at risk; decisions will be made on the protective measures needed to minimize risk. Seismic isolation is one protective measure showing promise to manage seismic risk.
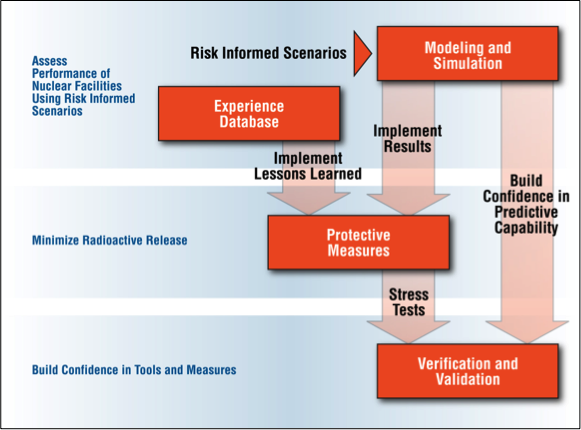
Future risk-informed process to minimize potential external hazard risk.
Seismic Isolation (SI) is gaining importance in the nuclear arena, especially after the Fukushima accident in 2011, which made the nuclear community even more aware that it must design and construct structures capable of withstanding large earthquakes (a previously alarming incident occurred in 2007; the Niigata Chuestu-Oki earthquake led to temporary shutdown of the Kashiwazaki-Kariwa NPP). Seismic base isolation has the potential to reduce horizontal earthquake loads for nuclear structures, systems, and components (SSCs). A substantial reduction in horizontal earthquake loading has the potential to increase the safety of nuclear SSCs by managing the risk associated with large seismic events. SI has been used for years in the non-nuclear commercial industry for buildings, bridges, liquid gas tanks, and offshore oil and gas platforms. In addition to SI systems for the current fleet of reactors, such as light water reactors (LWRs), there is a need for seismic isolation of nuclear facilities (both near-surface facilities and deeply embedded), related systems, and components such as diesel generators, reactor pressure vessels, steam generators, spent fuel pools, and critical response facilities. However, the seismic isolation solutions for LWRs (documented in ASCE-4) may not be appropriate for this purpose because the mass of many systems and components is relatively small and their geometry is very different.
The U.S. nuclear industry has not implemented SI to date. Therefore, a seismic isolation gap analysis meeting was convened on August 19, 2014, to determine progress on implementing SI in the U.S. nuclear industry, and what systems and components could benefit from SI. This working meeting included representatives from the Department of Energy (DOE), national laboratories, industry, Electric Power Research Institute (EPRI), and Nuclear Regulatory Commission (NRC) to discuss three SI topics: (1) general background on current SI progress in the United States, (2) limitations with implementing procedures outlined in ASCE-4 for SI solutions of entire nuclear power plants, and (3) potential SI solutions for systems and components, and gaps associated with developing standardized technologies, methods, and numerical tools for these solutions.
Seismic Isolation Need
Seismic hazard curves used in evaluating risk at nuclear facilities are continuously evolving as more information has been developed on seismic sources and seismic events. Moreover, additional research is performed to update attenuation relationships and characterize local site effects. Recent earthquake recordings at nuclear power plants sites have exceeded design basis values. These three recent earthquakes in the last 7 years are shown in Table 1. The seismic events were recorded at Kashiwazaki-Kariwa (TEPCO 1, 2007),1 Fukushima Daiichi and Fukushima Daini (TEPCO 2, March 2011),2 and North Anna (August 2011, detailed information provided in Virginia Electric and Power Company Memo).3

Peak recorded acceleration verses the design acceleration value.
The evolving nature of the seismic hazard curves and the requirement to reevaluate those curves every 10 years creates the possibility that a number of these seismic hazard curves will increase.4 Therefore, there is an opportunity for engineered solutions to manage this seismic uncertainty, and one solution is seismic isolation. SI has the potential to reduce horizontal earthquake loads for nuclear structures, systems, and components (SSCs). A substantial reduction in horizontal earthquake loading has the potential to increase the safety of nuclear SSCs by managing the risk associated with large seismic events. Installing horizontally flexible and vertically stiff seismic isolators between the superstructure and its foundation typically achieve isolation. Isolators serve two key functions: supporting gravity loads, and protecting the supported structure and its systems and components from the damaging effects of horizontal earthquake ground motion.
ASCE-4 provides standard procedures for providing robust seismic isolation systems for the entire NPP as shown in Figure 2. In addition to SI systems for LWRs (such as those shown in Figure 3), there is a need in the nuclear industry for seismic isolation of nuclear facility-related systems (both near surface facilities and deeply embedded facilities) and/or components (such as diesel generators, reactor pressure vessels, steam generators, spent fuel pools, and critical response facilities [such as FLEX support centers]).
FLEX is a strategy developed by the nuclear energy industry to implement the Nuclear Regulatory Commission (NRC)’s Fukushima task force recommendations5. FLEX provides backup electrical power and cooling capability if an extreme event impacts capability to maintain power and core cooling. One of the important aspects of FLEX is providing regional support centers that will provide emergency equipment to nuclear power plants during extreme events when necessary. FLEX support center locations are in Memphis, Tennessee and Phoenix, Arizona
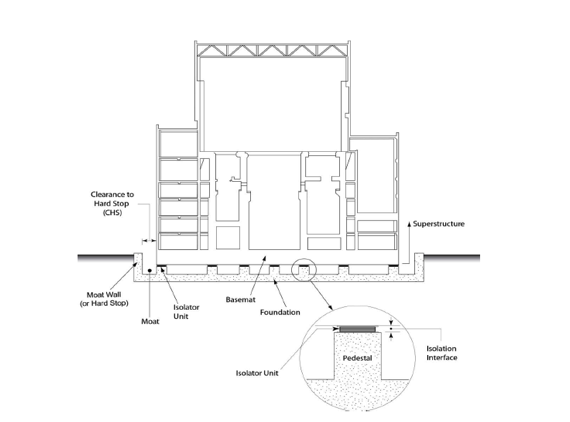
Proposed seismic isolation of LWR per ASCE-4.
The isolation solution shown in Figure 2 would not be cost effective for deeply embedded NPPs, since this would require over-excavation of the soil and installation of a moat system to accommodate the lateral displacement of the facility. Therefore, the likely benefit for deeply embedded structures would be systems and/or components internal to the NPP. For these systems and/or components, there may be a need to provide three-dimensional seismic isolation in addition to lateral SI. Due to the potential difference in the isolation environment (i.e., isolators may be located in the nuclear power plant instead of isolating the nuclear power plant) and the potential need to provide three-dimensional SI, it is necessary to provide required and improved numerical methods and consensus standards for SI of systems and/or components.
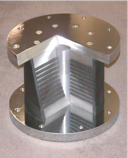
Seismic isolators: lead-rubber bearing (courtesy of DIS)
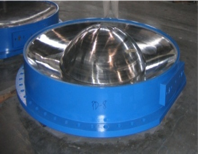
Seismic isolators: friction pendulum bearing (courtesy of EPS).
Seismic Isolation Gaps
The gaps identified in the working meeting fit into two areas: isolating the entire nuclear facility, and isolating systems and components. A systematic process was developed to determine the importance of each gap.
3.1 Entire Nuclear Facility
Hard Stop Cliff Edge Effects
The hard stop, as shown in Figure 2, is a concrete structure that limits the displacement of the installed seismic isolation system. The ASCE-4 committee has not addressed the potential cliff edge effects (and therefore unanalyzed damaged to the nuclear structures, systems, and/or components [SSCs]) during beyond design basis earthquake (BDBE). A cliff edge effect in a nuclear power plant is an instance of severely abnormal plant behavior caused by an abrupt transition from one plant status to another following a small deviation in a plant parameter, and thus a sudden large variation in plant conditions in response to a small variation in an input. So in the case of seismic isolation, the potential exists that during a large earthquake event the seismically isolated structure is appropriately decoupled from the ground motion; however, during the event a slightly larger displacement causes the structure to strike the hard stop. This impact could cause shock waves in the structure that damage critical components. This is an important parameter to consider in the SI application for a safe design.
Need: Medium
R&D would produce tools, methods, and technologies for addressing cliff edge effects. These would be implemented as guidance in industry consensus codes such as ASCE-4. Understanding of cliff edge effects (if any) and proposed solutions for design changes (if necessary) and demonstrating the reduction of in-structure nuclear facility response has potential to increase nuclear safety.
Hard Stop Requirements
Is the hard stop needed? Currently, ASCE-4 requires implementation of a hard stop to prevent displacement of the nuclear facility beyond what would cause tearing of the isolation system and catastrophic damage.
Need: High
Current state of knowledge is presented in ASCE-4, which requires implementation of the hard stop.
Seismic isolation methods and solutions for mitigating vertical seismic motion
SI of entire nuclear facility is standardized in a forthcoming version of ASCE-4. These are horizontal isolation systems. Vertical seismic response is an issue at some sites; there may be a need for vertical isolation. Currently, there is relatively little knowledge in the United States on designing robust vertical isolation. The need exists to understand the requirements, development methods, and solutions for vertical isolation.
Need: Medium
Vertical seismic response is an issue at some sites; a vertical isolation solution could manage the risk posed by large vertical ground motions.
Understanding margins in Seismic Analysis
Margins that may exist in seismic analysis are not clearly understood. Three recent earthquakes in the last 7 years have exceeded their design basis earthquake values (so damage to SSCs should have occurred), as shown in Table 1. However, seismic walk-downs at some of these plants indicate that very little damage occurred to safety class systems and components due to the seismic motion. Therefore, it is necessary to gather the applicable data to quantify the margins in current seismic analysis techniques (linear analysis) at nuclear power plants.
Need: High
Cost Benefit (Economic Viability) for SI of entire nuclear plant
To our knowledge, no one has performed a cost benefit analysis in the United States on an isolated versus non-isolated nuclear systems and components. A cost benefit analysis could provide valuable insight for venders interested in application of seismic isolation to manage seismic risk at their nuclear facilities.
Need: High
DOE and industry need to understand the cost benefit of implementing SI at NPPs.
Time Domain Methodology Requirements for Nonlinear Behavior
Industry needs a time domain approach that can handle nonlinear behavior, such as seismic isolation.
Need: Medium
Implementation of SI in Nuclear Facility Safety
The forthcoming version of ASCE 4 provides standardized language of implementation of SI for the entire nuclear facility. However, currently in the United States, SI related to nuclear facilities applications do not exist. After the Kashiwazaki-Kariwa earthquake in 2007, TEPCO installed seismically isolated emergency response buildings at all their NPP sites including Fukushima Daiichi (Hijikata 2012).6 These facilities provided valuable emergency response equipment to manage the nuclear reactor issues and allowed for continued onsite response.
Need: High
The consensus at the gap working meeting was that SI has the potential to manage seismic risk at NPP sites. One important comment from the meeting is there may be some reluctance in the nuclear community that first application of SI be the nuclear island. FLEX facilities, which support nuclear safety and other storage facilities, could be considered for SI application to demonstrate implementation of the technology in the United States nuclear safety arena.
3.2 Systems and Components
SI Solutions for Nuclear Systems and Components
Seismic isolation solutions for LWRs (documented in ASCE-4) may not be appropriate for isolation of systems and/or components because the mass of many systems and/or components is relatively small and their geometry is very different. Can SI solutions presented in ASCE-4 be scaled for implementation with systems and components, or is it necessary to develop new SI solutions?
Need: High
Deeply embedded NPP venders are likely to seek SI solutions (if needed) for systems and components. Currently, ASCE 4 does not address SI of systems and components; therefore, development of SI solutions for systems and components is necessary. Implementation of these solutions on consensus codes is necessary.
SI for Individual Components, Leading to Differential Displacement Issues
Differential displacement between the isolated and unisolated interface is a known issue that should be addressed in the design. It is also important to quantify the risk associated with this differential displacement.
Need: Medium
Model Validation and Uncertainty Quantification
SI numerical models must be verified and validated to provide confidence in predicted structural response. Nonlinear structural dynamics models are needed to accurately predict the behavior of seismic isolation systems. Uncertainty quantification is essential to accessing the range of validity for the model (based on assumptions) and experiments (based on operating conditions).
Need: Medium
Rocking Effect on Seismic Isolation of a Component (essential to understand for Integral SMR designs)
SI of certain NPP systems and components may create rocking loads that current isolation technologies (standardized in ASCE-4), were not designed to handle. SI solutions for deeply embedded structures will likely only be used for systems and components. Therefore, it is necessary to develop SI tools, methods, and solutions similar to what is currently in ASCE 4 for SI of the entire NPP.
Need: Medium
Depending on SI designs for systems and components, this may be important.
Results and Conclusions
Understanding the impact external hazards, such as flooding and earthquakes, can have on nuclear facilities and nuclear power plants is critical to deciding how to manage these hazards to expectable levels of risk. Seismic isolation offers the potential to manage nuclear power plant risk associated with large seismic events. An incremental step in implementing SI in the nuclear arena, prior to actually isolating some portion of a nuclear plant, could be an application for consolidated used fuel facilities, or the two FLEX locations in Memphis, Tennessee and Phoenix, Arizona, which are the two U.S. storage locations for spare diesel generators, pumps, etc., selected by industry in response to Fukushima.
The gaps and research and development needed to close these gaps are identified in Coleman and Sabharwall, 2014 (INL-14-33234).7This should be used to guide the future requirements for the research communities and industry. The gaps that are identified as most important are:
- SI solutions for nuclear systems and components
- Quantification of cost benefit (economic viability) for SI
- Understanding margins in seismic analysis
- Implementation of SI in nuclear facility
Implementation of seismic isolation at nuclear power plants and critical facilities has the potential to minimize risk at nuclear facilities associated with large seismic motions, and reduce the cost of construction. Therefore, it is necessary to perform research and development activities that focus on collaborative efforts between, industry, national laboratories, and universities to close the gaps that are identified.
Justin Coleman is a Civil/Structural Engineer at Idaho National Laboratory (INL). He performs seismic and structural dynamics research at INL and has a master’s degree in engineering structures and mechanics. Mr. Coleman’s background and expertise is seismic analysis of nuclear facilities and impact analysis of spent fuel casks. Much of the impact analysis work is performed using explicit finite element analysis codes. He has performed linear and nonlinear seismic soil-structure interaction (SSI) analysis for safety-related nuclear structures and is currently working to develop advanced nonlinear seismic soil-structure interaction analysis methodologies. His research interests include nonlinear SSI analysis, advanced seismic PRA, seismic protective systems, spend fuel transportation and storage, and beyond design basis threats to nuclear structures. He serves on the ASCE 4 and 43 where he is the lead author of Chapter 3, “Modeling of Structures,” and led the effort to write Appendix B, “Nonlinear Time Domain Soil-Structure Interaction Analysis,” in the forthcoming edition of ASCE 4. Mr. Coleman has authored numerous reports on impact analysis of spent fuel casks and nuclear fuel, and seismic analysis.
Dr. Piyush Sabharwall is a staff research scientist in Nuclear System Design and Analysis Division at Idaho National Laboratory (INL). He has a premier role in the development of very high temperature nuclear reactor technologies that are integral to the Department of Energy (DOE) strategic plans for the sustained advances in nuclear energy. Dr. Sabharwall has expertise in heat transfer, fluid mechanics, thermal design, thermodynamics, and nuclear safety analyses. Over the last few years, he has been researching on high temperature heat exchanger design & optimization, system integrations & power conversion systems and safety and reliability for Advanced Reactor Concepts. He obtained his Masters in Nuclear Engineering with a minor in Mechanical Engineering from Oregon State University and further pursued his Doctorate in Nuclear Engineering from University of Idaho. He has authored over 75 publications including journal articles, conference proceedings, technical abstracts, magazines and technical reports and has acted as a reviewer for journal and conference proceedings. He has been a significant catalyst for international and national research partnerships in the field of thermal hydraulics (heat and mass transfer, fluid mechanics, two phase flow, computational multiphase flow technology) for both nuclear and mechanical industries. In 2011 he received the New Faces of Engineering ASME National Award on the basis of contributions stemming from experiments and research and development effort in the area of thermal hydraulics and in 2013 he was awarded the ANS Young Member Excellence National Award.
Verification Requirements for a Nuclear Agreement with Iran
Negotiations are currently underway with Iran regarding their nuclear program; as a result, one of the main questions for U.S. government policymakers is what monitoring and verification measures and tools will be required by the United States, its allies, and the International Atomic Energy Agency (IAEA) to ensure Iran’s nuclear program remains peaceful.
To answer this question, the Federation of American Scientists (FAS) convened a non-partisan, independent task force to examine the technical and policy requirements to adequately verify a comprehensive or other sustained nuclear agreement with Iran. Through various methods, the task force interviewed or met with over 70 experts from various technical and policy disciplines and compiled the results in the new report, “Verification Requirements for a Nuclear Agreement with Iran.” Authored by task force leaders Christopher Bidwell, Orde Kittrie, John Lauder and Harvey Rishikof, the report outlines nine recommendations for U.S. policymakers relating to a successful monitoring and verification agreement with Iran. They are as follows:
Six Elements of an Effective Agreement
1. The agreement should require Iran to provide, prior to the next phase of sanctions relief, a comprehensive declaration that is correct and complete concerning all aspects of its nuclear program both current and past.
2. The agreement should provide the IAEA, for the duration of the agreement, access without delay to all sites, equipment, persons and documents requested by the IAEA, as currently required by UN Security Council Resolution 1929.
3. The agreement should provide that any material acts of non-cooperation with inspectors are a violation of the agreement.
4. The agreement should provide for the establishment of a consultative commission, which should be designed and operate in ways to maximize its effectiveness in addressing disputes and, if possible, building a culture of compliance within Iran.
5. The agreement should provide that all Iranian acquisition of sensitive items for its post-agreement licit nuclear program, and all acquisition of sensitive items that could be used in a post-agreement illicit nuclear program, must take place through a designated transparent channel.
6. The agreement should include provisions designed to preclude Iran from outsourcing key parts of its nuclear weapons program to a foreign country such as North Korea.
Three Proposed U.S. Government Actions to Facilitate Effective Implementation of an Agreement
1. The U.S. Government should enhance its relevant monitoring capabilities, invest resources in monitoring the Iran agreement, and structure its assessment and reporting of any Iranian noncompliance so as to maximize the chances that significant anomalies will come to the fore and not be overlooked or considered de minimis.
2. The U.S. Government and its allies should maintain the current sanctions regime architecture so that it can be ratcheted up incrementally in order to deter and respond commensurately to any Iranian non-compliance with the agreement.
3. The U.S. Government should establish a joint congressional/executive branch commission to monitor compliance with the agreement, similar to Congress having created the Commission on Security and Cooperation in Europe to monitor the implementation of the 1975 Helsinki Accords.