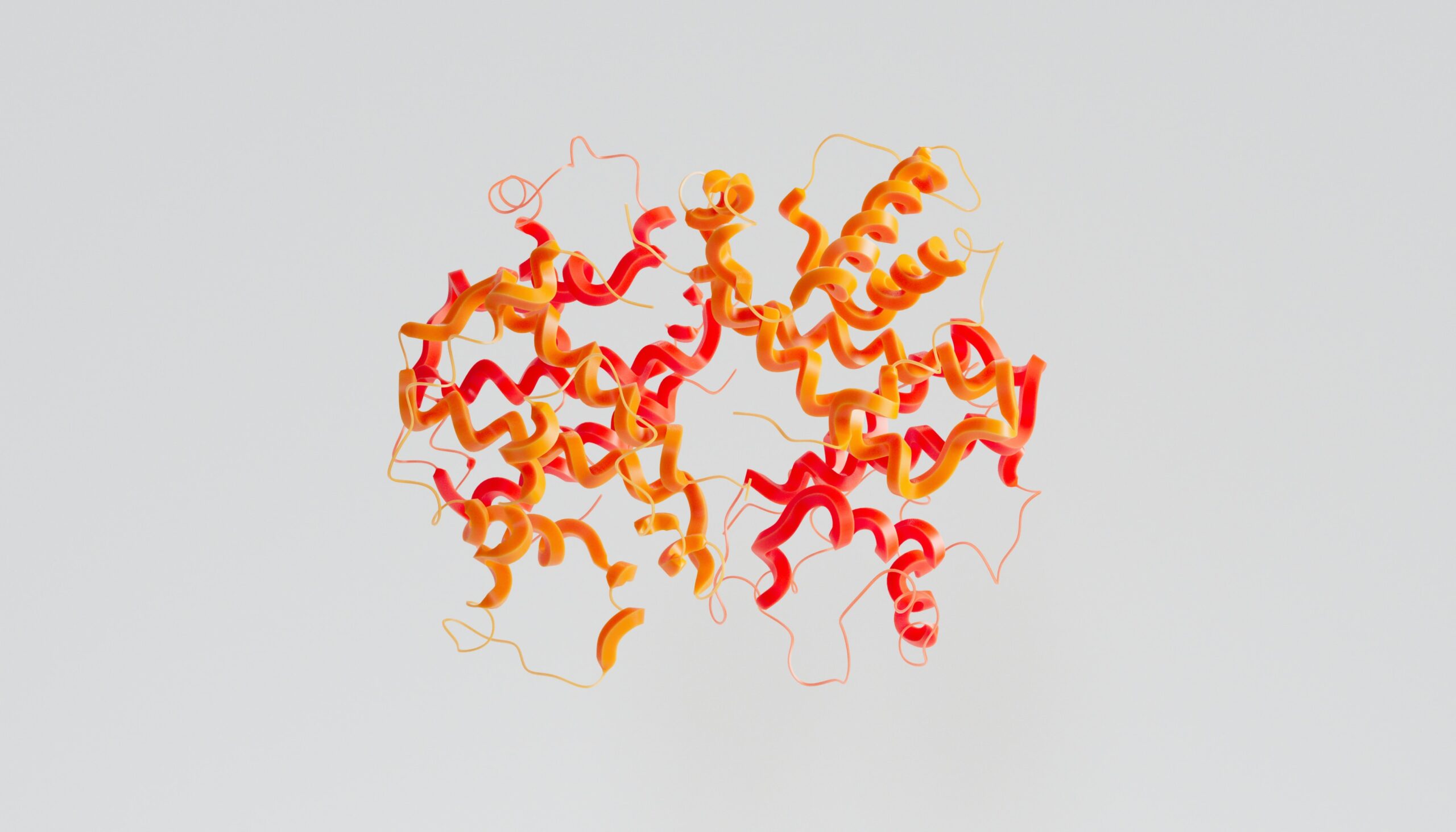
A “Focused Research Organization” to Develop a Modular and Scalable Platform for Human Molecular Monitoring
Wearable health electronics are now ubiquitous, but continuous molecular monitoring is only widely available for glucose. Decades of research have expanded continuous monitoring to other molecules, but these techniques are restricted to research labs and remain disconnected from daily human use. We propose a platform to translate and distribute these emerging technologies, enabling the mapping of the time-varying human metabolome and the design of closed-loop devices for personalized health.
Problem Statement
Humans are the best model organisms for humans, yet we have few tools to study human biochemistry in situ and in real time. The Human Metabolome Database lists ~20,000 detected compounds, of which only ~3,000 have been quantified. Even fewer of these biomolecules have been studied with time resolution in longitudinal human studies.
Cardiovascular, metabolic/endocrine and drug pharmacokinetic phenomena are driven by biomolecules varying on the time scale of seconds to minutes to hours, impacting behavior and well-being on similar time scales. Currently, the only way to measure these molecules is through laboratory testing, which is obtrusive to daily life. Moreover, laboratory testing cannot be conducted at the frequency necessary to capture all of these variations.
In contrast, wearable monitors are user-friendly and enable continuous measurements at the correct time scale. These monitors require specially engineered biosensors, since there are a limited number of naturally-occurring enzymes that generate continuous, time-varying electrical signals like those used by continuous glucose monitors, which are currently the only commercially available device of this kind. However, most labs that pioneer biosensing strategies do not develop human-compatible devices and vice versa, creating a chasm between these two areas of research and development.
Project Concept
This project aims to achieve minimally invasive continuous monitoring of 100+ analytes in the human body and deliver devices to researchers. This project will develop a medical device testbed that can use the myriad biosensing strategies pursued by academic labs to develop devices for human experiments. Our technical approach combines synthetic ion channels and conformational switches coupled with a CMOS array to assess many analytes in parallel. We already have access to customized fabrication techniques, and the cost and barriers in designing and manufacturing proteins and silicon sensors are trending downwards.
The project will progress through four interdependent stages:
- Survey and prioritize metabolic, hormone, and immune targets that provide the greatest explanatory power for well-being. Select biosensor and transduction systems reported in the literature to interface with our platform.
- Develop an integrated circuit functionalized with biosensors for parallel multi-analyte sensing packaged in a wearable form factor. Test devices in humans to validate against conventional blood sample analyses.
- Specify and share validated devices in bulk to catalyze large-scale human field research.
- Curate a time-varying human metabolome.
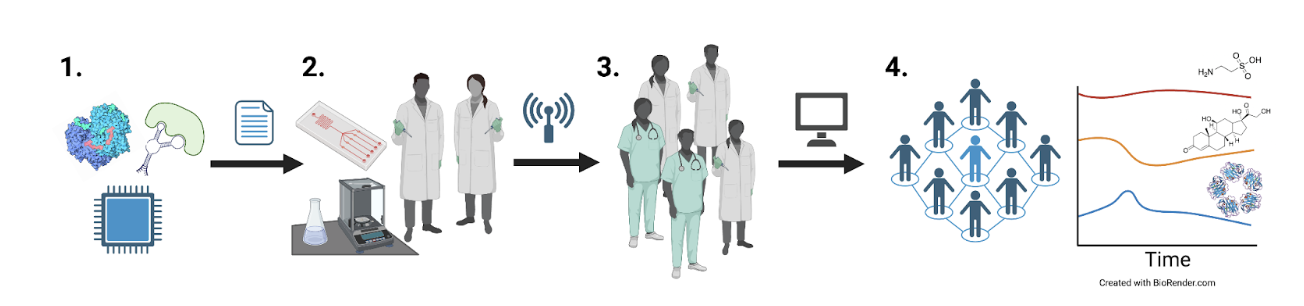
The project will progress through four interdependent stages.
What is a Focused Research Organization?
Focused Research Organizations (FROs) are time-limited mission-focused research teams organized like a startup to tackle a specific mid-scale science or technology challenge. FRO projects seek to produce transformative new tools, technologies, processes, or datasets that serve as public goods, creating new capabilities for the research community with the goal of accelerating scientific and technological progress more broadly. Crucially, FRO projects are those that often fall between the cracks left by existing research funding sources due to conflicting incentives, processes, mission, or culture. There are likely a large range of project concepts for which agencies could leverage FRO-style entities to achieve their mission and advance scientific progress.
This project suits a FRO-style model because the four research stages require tight feedback loops and standardization and the medical device industry is not incentivized to pursue this kind of research. Private companies typically focus on a handful of molecules most relevant to diabetes care, taking advantage of proven biosensors and predictable insurance reimbursement. A stand-alone, non-profit institute is best suited to standardize and derisk experiments on molecular monitoring to catalyze the formation of a consortium of experimenters. Just as the nonprofit AddGene has standardized and democratized access to genetic material, we seek to develop the analog institution for medical devices.
How This Project Will Benefit Scientific Progress
Human physiology is currently a poorly explored, high-dimensional space, and scientific labs lack the tools to measure human biochemistry over time. The devices developed through this project will first help scientists study specific questions in domains such as disease etiology, human behavior, and drug discovery. Study validity will be enhanced by providing additional molecular time courses which will clarify the relationships between conditions, specific biomarkers, and interventions. Next, these studies will enable the development of a human molecular atlas, similar to the Human Metabolome Database but with time resolution. This database could act as a powerful tool for developing nuanced models of human physiology to uncover previously overlooked phenomena. Finally, similar devices will ultimately become accessible as consumer health products, enabling the next generation of personalized health.
Key Contacts
Authors
- Anand K. Muthusamy (amuthusa@caltech.edu) and
- David Garrett (dgarrett@caltech.edu), Caltech
Referrers
- Matt Hourihan, Federation of American Scientists, mhourihan@fas.org
- Alice Wu, Federation of American Scientists, awu@fas.org
Learn more about FROs, and see our full library of FRO project proposals here.
The overarching strategy involves converting biochemical interactions into a time-varying electrical signal and isolating specific interactions into separate channels on a CMOS chip. We envision a universal platform that can accept the myriad of biosensors from labs worldwide. Advances in synthetic biology and protein engineering have unlocked various modes of biosensing. Oxidoreductases, like glucose oxidase used in CGMs, provide a direct conversion of the local ligand concentration to an electrical current. Recent advances in deep learning protein engineering have shown proof of concept for the de novo design of enzymes, but some classes of biomolecules such as nucleic acids and proteins would be out of reach for enzymatic detection. In turn, we can either mimic nature’s ion channels or create our own electronic transduction systems. Aptamers are amenable to high-throughput evolution to bind a variety of targets and provide a hairpin motion that can move redox probes to and away from an electrode surface (cf. example of a cortisol sensor). Recent advances in DNA origami have enabled synthetic, self-assembling nanopores whose current can be modulated by the binding of other biomolecules. Both biological and solid-state nanopores are increasingly used in commercial scientific equipment, providing a tailwind for medical devices.
All of these biosensor and transduction systems can be integrated into field-effect transistors (FETs). Even strategies involving membrane proteins can now be interfaced with transistors thanks to organic electrochemical transistors with supported lipid bilayers harboring ion channels and nanopores. Modern complementary metal oxide semiconductor (CMOS) processes allow us to parallelize many sensors onto a single device at a low per-unit cost. Each sensor consists of a FET sensing unit, where the presence of molecules of interest induces a surface potential in the FET gate. This modulates the current in the FET, which is then measured with read-out circuitry. Each sensor’s current is then digitized and used as an indicator of molecular concentrations. Since relatively few channels are required (~100s) compared to conventional CMOS devices, coarser fabrication processes could be used (e.g. 130 nm) which would reduce cost and time while still allowing for a compact active area (~mm2).
The CMOS device will be packaged in a wearable form factor miniaturized for on-body measurements using a battery and wireless data transmission to a smartphone (e.g. using Bluetooth low-energy). We will draw interstitial fluid via reverse iontophoresis to reach the CMOS chip sitting next to the skin surface. Since different analytes have different rates of variation and concentration ranges, we will model the appropriate temporal resolution and SNR we can achieve. This device form factor and reverse iontophoresis method has been validated for ~mM glucose measurements with ~min resolution. In contrast, hormones may be present at ~6 orders of magnitude lower concentrations but only vary over days-weeks. Using our CMOS configuration, different channels can be read out individually. To efficiently capture these changes, different sampling rates will be used to ensure we capture their peaks and troughs while minimizing energy consumption. For analytes with lower baseline concentrations, techniques like averaging (over multiple samples and CMOS channels) may be required to improve sensitivity. The mean absolute relative difference (MARD) will be computed against blood LC-MS ground truth.
We are in a position where our data science methods outpace quality datasets. For example, geometric deep learning is useful for mapping interactions in a metabolome, and long short-term memory is well-established for analyzing and predicting time series data. These data can become actionable in a closed-loop device where a biosensing event triggers an alert or pharmacological intervention as in an artificial pancreas. Here again, control theory approaches to physiology are sufficiently mature.